"Science cannot tell theology how to construct a doctrine of creation, but you can't construct a doctrine of creation without taking account of the age of the universe and the evolutionary character of cosmic history." -John Polkinghorne
Out there in space, whether we look with our eyes or with a telescope -- a far more powerful version of our eyes -- we find that the Universe is full of stars, galaxies, clusters, and luminous objects everywhere we look.
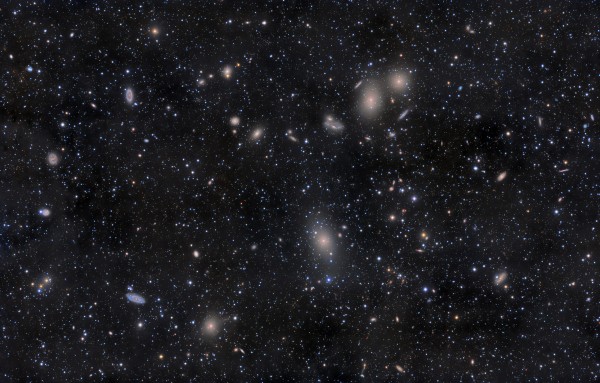
But if we look in different wavelengths of light than what our eyes can see, we're going to see the Universe in a whole new light, literally. X-rays show us where black holes, neutron stars, and ultra-hot gas is, ultraviolet light shows us the hottest, youngest stars in the Universe, near infrared shows us cooler stars and is transparent to all but the hottest neutral atoms that normally block visible light, while far infrared shows us warm and cool gas and dust, including the locations of future stars.
But if you look in the microwave part of the spectrum, you see something that is, perhaps, a little unexpected. You see, if you look in, say, the infrared, what you'll see is completely dominated by the local group: our galaxy, the stars in it, and the nearest galaxies to us.
If you're clever enough to subtract out those local sources from your sky map, what you'll find is a slew of point sources that show off the structure of the Universe: galaxies, clusters and filaments lying beyond our own galactic neighborhood. When you look at a picture like the one below, we are looking at a map of the large-scale structure of the cosmos.
But what if we look in microwave wavelengths? Instead of seeing this rich structure that shows us point sources, galaxies, black holes, gas, dust, or something like this, what we instead see -- once we subtract our galaxy out -- is this.
Believe it or not, that's the picture of our Universe in microwave wavelengths. The microwave sky shows us the same 2.725 Kelvin temperature radiation in all directions in the sky, a leftover relic from the hot Big Bang when our Universe was just 0.0027% of its present age! For perspective, if the Universe were scaled to be exactly one year long, so that right now is 11:59 PM on December 31st, this is a picture of what the Universe looked like at 12:14 AM on January 1st!
And this is a picture of the fluctuations in the cosmic microwave background, or the temperature differences in different regions of the sky. Just a few hundred microKelvin separate the hottest regions from the coldest here, with the coldest (bluest) regions actually showing us the regions of space from 13.82 billion years ago that have slightly more matter (and hence a deeper gravitational well for the photons to climb out of, making them appear colder) than average, while the reddest (hottest) regions are the least dense regions.
That's what we see when we look at the Universe in microwave wavelengths: the Cosmic Microwave Background (CMB). But that was all background: here are 5 facts about the CMB that you might not know, even if you're a professional astrophysicist!
1.) The Cosmic Microwave Background actually extends far into the infrared and radio spectrum!
That number that corresponds to the temperature of the CMB -- 2.725 K -- is the photon energy (converted into a temperature via Boltzmann's constant) of the peak of this radiation. But the photons in the Universe come from a thermal bath, where matter, radiation and everything else from the young Universe was constantly colliding with every other particle it saw, exchanging energy and thermalizing. This produces a very special spectrum to the radiation, known as a blackbody spectrum. Every photon in the Universe cools as the Universe expands and stretches the wavelength of each one, but the shape of this spectrum is preserved!
The photons may still peak in the microwave, but they play a role in the infrared -- particularly at wavelengths longer than about 300 microns -- throughout the entirety of the microwave range and all the way into the radio, where wavelengths are the size of your hand!
2.) The Cosmic Microwave Background is a "surface" over 100,000 light-years thick!
The photons of the CMB smack into free electrons and protons all the time, whenever they see one. Once the Universe cools enough so that the atoms become neutral, the vast majority of these photons now stream freely for the next 13.8 billion years, until they run into something like our detectors. But the Universe didn't become neutral all at once; nuclei and electrons have been finding one another for hundreds of thousands of years, only to be blasted apart by a high-enough energy photon! When enough time has passed and the photon background has cooled enough, collisions like this become more and more rare, and eventually, the Universe is cool enough so that photons will free-stream all the way to your eye. That's why the CMB is sometimes called the "surface of last scattering."
Only, it's not quite a surface in any direction: it takes somewhere around 117,000 light years for the Universe to go from a completely ionized to a completely neutral state, and the photons we see come from all sorts of different points along the way in every direction.
But there is something remarkable about the CMB we see today...
3.) It only became neutral when it did because of a curiosity of chemistry!
The simplified picture I presented to you -- a high-energy photon background cooling as the Universe expands -- would explain why atoms became neutral and the CMB appears as a rough "surface," despite having a finite thickness. But think about it: every time you get a neutral atom forming, it emits a photon, which can then be absorbed by another neutral atom, ionizing it again! Sure, eventually the Universe will have expanded enough so that we get our surface of last scattering, but that surface would have been a lot thicker than 117,000 light-years if this were the dominant effect.
In fact, there's another effect that's much more important!
When the electrons in hydrogen and helium -- which make up 99.999999% of the Universe at this point -- transition to the ground state, they don't just emit one photon, but two! This makes all the difference; rather than traveling until they hit another atom, kicking it up into a higher-energy state, you'd need two photons to hit at the exact same time, something that's so rare it effectively never happens in physics! It's only for the existence of this atomic transition that the Universe becomes neutral as quickly as it does.
Some day, far into the future, the cold spots will grow into regions richer in stars, galaxies and clusters (on average), while the hot spots will grow into underdense regions, with below-average numbers of all of those things. But...
4.) The hot-and-cold spots you see in the CMB today are completely unrelated to all the structure in the Universe!
Because the last-scattering surface has a thickness of about 117,000 light-years, that means with the passage of time, that structure changes! In fact, the last-scattering surface will look completely different 117,000 years from now, if we're still around to see it. The structure we see in the Universe has evolved from a huge cosmic web of initial seed fluctuations, spread out all across the Universe. But the structure we see here is related to what the CMB looked like billions of years ago, not the CMB we see today!
Sure, as far we can tell, the CMB would have looked different only in detail and distribution; the spectrum of fluctuations would be indistinguishable regardless of when we look.
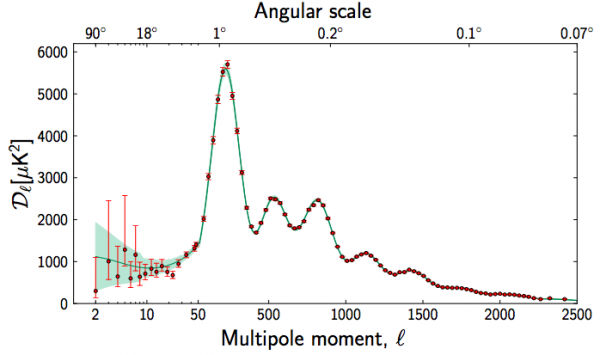
But this spectrum tells us one final, very, very interesting thing...
5.) There's a lower-limit to the size of gravitationally-bound structure in the Universe!
Due to the presence of photons in the early Universe, initially large fluctuations get washed out over time, and become smaller and smaller in magnitude on smaller and smaller scales. The lowest mass that can exist in its own bound structure at this time is on the order of a few hundred-thousand solar masses. If all of these were in the form of normal matter...
We'd get globular clusters, or collections of around 100,000 stars and up! We do get plenty of them, but remember, the Universe is also full of dark matter. And so we'd expect to also get structures dominated by this dark matter, where -- after a little burst of star formation -- only a tiny amount of stars remain.
The Universe, according to our understanding of the physics going all the way back to when the Universe is just a few hundred thousand years old, should be filled with not just globular clusters, but also tiny, dark-matter-dominated structures with just around 1,000 stars or even fewer! Good thing it's 2013 and not 2005, because we've found them!
With just around 1,000 stars in a structure containing 600,000 solar masses (mostly dark matter, obviously), Segue 1 was the first one discovered, and now there are others! This is right around what's predicted, and it tells us that there are likely hundreds to tens-of-thousands around every galaxy.
All of that comes from the physics underlying the cosmic microwave background, and now you know!
- Log in to post comments
@Ethan -- your fifth image (the 53 GHz "flat" COBE plot) is a bit of a cheat. In fact, the "raw" COBE data is dominated by the dipole induced by our motion through the universe (http://apod.nasa.gov/apod/ap090906.html). The data does not show "the same 2.725 Kelvin temperature radiation in all directions," but is rather warmer in the direction of our motion, and cooler opposite. Your fifth figure is the result of correcting for ("boosting") that dipole motion.
This fact is at least as interesting as the perfect blackbody spectrum: There exists a natural reference frame in which the Universe is at rest, and we can measure our own relative motion through it.
Michael,
That is true; maybe I should have made the list 6 items long and put that in!
There is no chemistry in #3. No bonds = no chemistry
I'm not seeing the relevance of point #3, which presumably has to do with selection rules in atomic state transitions, in the CMB story. True, in the absence of collisions you need two photons to transition between the 1s and 2s states of a hydrogen-like atom, or from 1s^2 to 1s2s in neutral helium, because with a single photon process and no collisions your allowed values of ΔL are +1 and -1. But that doesn't help you with photons that are energetic enough to ionize your atom, because the selection rules don't apply there (the electron can carry off the angular momentum of the photon). A 2s hydrogen atom is every bit as neutral as a 1s hydrogen atom; it's just not in the ground state. Furthermore, the "no collisions" caveat is essential, because when you do have a collision the other body can absorb the angular momentum quantum. We know this happens because the Lyman alpha line, and its equivalent for He+, are observed.
"But that doesn’t help you with photons that are energetic enough to ionize your atom"
Which is Ethan's point.
As with pair production, where two photons are created, when you share out your energy into two photons, the resulting photons become unable to keep the reaction going on their own.
Hence you need a photon and atom density high enough that the chance of collision within the relaxation time of the excited atom (which is shorter for more energetic states, which only require two photons to manage to ionize) is higher than 1.
When it's less than 1, you get a logarithmic decay of the ionising potential for the universe.
And because it bisects the energy, it cuts down much quicker than the linear subtraction of energetic free electrons radiating when captured again.
As with pair production, where two photons are created, when you share out your energy into two photons, the resulting photons become unable to keep the reaction going on their own.
But that assumes that you actually require two or more photons to make a transition. Which is true for an ns -> n's transition, but there are some cases where this is not true. There is one in the diagram Ethan shows: going from the state labeled 1s5p (I suspect this is a typo and should be 1s2p) to 1s^2 in the neutral helium atom. Transitions like that one, or np -> n's in a hydrogen-like atom, can be done with one photon because ΔL = -1, as the figure shows. Likewise with capturing a free electron: as long as you have ΔL of +1 or -1, you can do it by emitting a single photon. It may be the case that most electron captures involve different values of ΔL, but Ethan does not say this in the post.
What's probably more important is that at some point your population of ionizing photons is depleted due to redshift. Once the mean free path of ionizing photons becomes long enough, some fraction of the photons emitted when a nucleus captured an electron are no longer energetic enough in the frame of an atom they encounter to ionize that atom. So instead, if it's at one of the right energies, it kicks the electron from an s state to a p state, or from a p state to either an s state or a d state. You also need a long enough mean free path for the atoms so that they don't de-excite by collision, where as I said the other atom can carry off the extra angular momentum. Only then do multi-photon pathways become important.
Doesn't exist any Big Bang!!!!!
Lucy Haye
Eric Lund,
It isn't that you require the transition to occur between the different ns states (and 2s --> 1s is the dominant process, although others may play a role at up to the ~1% level), but that when a 2s --> 1s transition happens, there's no substantial back-reaction.
Yes, the 2p --> 1s transition is more common than the 2s --> 1s, but it's not a 100%/0% split. So even if this occurred just one out of a hundred times, or one out of a million, eventually, it will occur, and when it does, you've just made a net gain of one neutral atom. And this occurs frequently enough that this is by far the dominant way that neutral atoms form in the Universe during recombination.
For a little more on the theoretical background of this, you might want to check out the Lamb Shift: http://en.wikipedia.org/wiki/Lamb_shift
"But that assumes that you actually require two or more photons to make a transition. "
Which is true for all photons that were emitted by a transition that emits two photons. Every time those transitions occur, those photons are no longer capable of re-exciting another atom on their own. You can throw in a multiplier to account for the proportion of interactions that involve those transitions, and it won't change that you're losing potentially-ionizing energy at a logarithmic rate.
Obviously the universe has to cool to a certain point for this effect to matter, and obviously the universe would have eventually cooled to the point where the atoms would have become neutral anyway. However that point would have been much later if all the helium and hydrogen transitions emitted single photons. This is the point.
Ethan: I didn't "get" fact 5, and wonder if the dark-matter inference sounds a bit of a stretch. Segue1 appears to contain a lot of "primordial" stars and hardly any neutral hydrogen. Couldn't the dark matter be non-luminous ordinary matter?
And what about fact 6? You hinted at it in fact1. The CMBR has redshifted by a factor of about a thousand. A long-wavelength photon has less energy than a short-wavelength photon. So, given that conservation of energy holds good as far as we know: where's the energy gone?
@Michael Kelsey re #1: I like the "natural" reference frame. Particularly since the universe is as absolute as it gets!
Getting scooped by Ethan is the best. :)
If someone might explain this to me: what is the meaning of saying that the surface of last scattering is 100,000 lightyears "thick"? Is that the same as saying it took 100,000 years from the beginning of last-scattering to the end of last-scattering? So it's 100,000 YEARS thick? Is this a stupid question?
I had not heard of the dipole that Michael mentions in his comment. I thought that Relativity ruled out all fixed reference frames.
I would like to learn more about this. Would you consider doing a post on it or putting up some layman level links?
I understood small parts of this. The real sweet spot for me was #4 where I learned an answer to a question I had for a long time. The CMB pattern does not correspond to large scale structures in the universe today. That gave me closure. Thanks.
@StellarAsh re #14: google on CMB dipole anisotropy. Relativity doesn't rule out a "universal reference frame" at all. Remember that Einstein's greatest blunder involved a static universe that would have provided a different sort of universal reference frame.
The CMB Dipole is definitely a good topic for down the road here. I've got something else I want to write about today, but I'll definitely put it in the ol' mental incubator. When it's fully baked, maybe in a week or two, you'll get your wish.
Ethan, you MONSTER.
You do NOT confuse an incubator with an oven!
(I was in an incubator for two months. Gas mark 3, I think... :-))
StellarAsh:
The Relativity Principle that Einstein's theories are based on states that the laws of physics have the same mathematical formulation in any reference frame (non-accelerating in the Special case, any reference frame at all in General).
You may have heard this referred to as "There is no 'privileged' reference frame", but this is a very specific meaning of "privileged" which means that there isn't any singular frame where the laws of physics only have to apply to it.
The classic example is the conundrum with Maxwell's equations which said the speed of light in a vacuum was constant, which conflicted with Newtonian mechanics which said that velocities add linearly. The Aether was proposed as the singular reference frame in which Maxwell's equations would apply as written. You would only ever get the right answer for an experiment by accounting for your motion relative to the Aether.
In the Relativistic world, Maxwell's equations apply everywhere, the speed of light is constant relative to you, and you can always consider the reference frame in which you conduct your experiment to be motionless (or moving at a fixed velocity) and it makes no difference.
There can of course still be "special" reference frames in the more human sense of being of particular interest or usefulness. That's fine. As long as we are able to consider ourselves to be at rest, and the rest of the universe moving relative to us, and still get the same answers. Which we are. So everything is cool.
This is a little off the subject, but supposedly in the MB they can see the evidence of the so called "dark flow" of a large number of galaxies toward the visible edge of the universe, any ideas of what could be the cause?
How do we know that the 600k solar mass cluster contains mostly dark matter and not, say, a supermassive black hole and 1000 stars? Is this supported by other unrelated observations or just assumed because it aligns with a model?
The motions of the visible stars will show the concentration of matter radially through the cluster. If the matter does not constrain itself to a virtual point then it isn't a supermassive black hole
Which is to say that why yes it "just" aligns with a model, and doesn't align with others.
Alignment with a model proves nothing alone. Observational evidence ruling out all other known models is much more compelling. Ethan has written a number of convincing articles explaining why the existence of DM is the only known explanation for a large set of independent observations. This is the essence of my question. Are their other plausible explanations that cannot yet be discarded through observational evidence?
With a statement like "mostly dark matter, obviously", I would assume that the answer is "yes" to those familiar with the literature, but experience has taught me that any assertion connected to "obviously" is worth additional questioning.
It proves that the model is good enough to describe reality.
And if observational evidence is against a supermassive black hole, the that proves your model incorrect.
Alignment (or not) with a model is all you ever "prove" in science. But "are there other models that aren't ruled out" is a fair question. They observed the velocities of the stars in the mini-galaxy, and that rules out things that don't act like a diffuse source of gravity.
It would be interesting if any of the modified gravity theories have been applied to this object. I wouldn't be too surprised if they work since there are plenty of other galaxies where they can't be ruled out, but on the other hand, maybe it doesn't track the changing dark/visible matter ratio. DM has an explanation for why as total (apparent) mass gets smaller, the dark/visible ratio gets bigger.
Polkinghorn, that buffoon, really? Oy.
Else, nice article!
"...the regions of space from 13.82 billion years ago that have slightly more matter (and hence a deeper gravitational well for the photons to climb out of, making them appear colder) than average, while the reddest (hottest) regions are the least dense regions."
The bit in parenthesis was very enlightening. good post sir!
2014 is the 50th anniversary of the discovery of cosmic microwave background radiation, that pay foundation to the Big Bang theory. Arno Allan Penzias and Robert Wilson were the scientists behind the find.
prof premraj pushpakaran
http://scholar.google.co.in/citations?user=Ur9mg_AAAAAJ&hl=en