"The discovery that young galaxies are so unexpectedly bright--if you look for this distinctive green light--will dramatically change and improve the way that we study Galaxy formation throughout the history of the Universe." -Matthew Malkan
Galaxies come in many different colors today: white, blue or red, mostly, depending on the populations of stars inside. But in a very rare set of circumstances, there can be green emissions as well, due mostly to the emission lines of doubly-ionized oxygen. The problem is we only see these in extraordinarily hot regions of the Universe, where ultra-hot stars or extremely unusual ultraviolet processes are found.
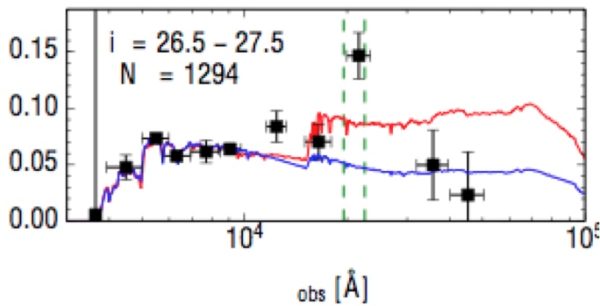
Yet by looking at the farthest star-forming galaxies available, two scientists broke the light up into components and found something shocking: this green emission feature was found to be incredibly strong in every one of thousands of galaxies that fit the criteria. It leads to a huge mystery: how do these ultra-hot stars form in the first place? Is it metallicity? Higher stellar masses? Or a top-heavy initial mass function?
- Log in to post comments
Question. How can the earliest galaxies be oxygen rich? If BB created mostly H and He and tiny amount of rest, wouldn't you need at least several generation of stars going nova and releasing oxygen before oxygen rich galaxies could form?
Haven't read the forbes article, but here's my interpretation of what you're taking about.
in the early universe there were several large stars. Large stars go boom REALLY quickly. Like millions of years. A change in the 4th sig fig in the age of the universe. And when the star goes boom, most of it is thrown away.
If there are several nearby stars or the gas cloud is still quite dense, the elements thrown out will be mixed in very quickly in the closest stars who may not be as big but burn a lot longer, or be mixed in to the gas forming the next stars to be born nearby. Which is still in the early millions of years of star formation. Like REALLY early still.
And Oxygen is quite a common component of stellar atmospheres (compared to the metallicity of normal stars running their main sequence) when they go boom. And a lot of that is thrown out, heavier elements being deeper down in the stellar shell formation.
So you'll have many stars artificially enriched because they were near enough to a really BIG star. And most of the stars won't be near enough these (less frequent) really big stars and hence won't be enriched.
The effect therefore is very clumpy Oxygen densities, where on average it's VERY poor, but because there hasn't been enough time to mix things around much, what little there is is still in small volumes of space.
@1: You can find 1.5-page summary of the research at: phys.org/pdf403163684.pdf
It sounds from that like they're talking about stars that formed in the first 2 billion years. Since IIRC stars started forming when the universe was 200 million years old, there's a good billion and a half years' worth of second-generation stars included in that range of data.
@Sinisa Lazarek #1,
I thought that this cool overview of the periodic table and its origin was slightly relevant:
https://pbs.twimg.com/media/C1mhrzvXAAEwh3N.jpg:large
Not sure if Ethan has ever used it already is some post.
@4: interesting. Though it somewhat arbitrarily cuts off at 92, when the most likely explanation for why we don't see higher-z elements in nature is because of theiir half-life, not because they weren't produced.
Nobody is sure what the stability limit is, but my guess is supernovae produce everything up to that limit. The higher-z stuff just decays with little trace.
Found what was confusing me. The original research is looking at galaxies at z=3 .. or about 2 billion years after BB, and found that a lot of them have a greenish glow associated with oxygen. All good.
Then Ethan said that it might be dwarf galaxies like these that re-ionized the Universe. But re-ionization happened 500 million to 1 billion y. after BB. While there should be plenty of time to get enough oxygen to glow green after 2 billion years, I wasn't sure there is enough time for them to start glowing green just after i.e. 700 million years. Pretty active times it would seem.
As for the periodic table... funny enough... just couple of days ago I watched a sixty symbols episode on youtube where they covered r and s-process in stars. And they showed the very same type of table :) dejavu
@eric
the reason it cuts after 92 is that the rest are man made and not found naturally in the universe.
I'm aware of that. But as I said, the likely reason they aren't found is their half-lives make it unlikely humans will find them, it's (IMO) not because nature doesn't produce them. There is no physical reason or theoretical basis for thinking the r-process shuts off at whenever [target Z] + [number of captured neutrons] = 92.
" it’s (IMO) not because nature doesn’t produce them."
yeah of course. they have very short half lives, so even if some are produced.. they decay almost instantly.
though.. just checked.. the other table does include two more (U and Np) as produced in supernovae before man-made ones start
Some have quite long half lives, SL. Just not long enough to last from the big bang/high-z era in any usefully measured ammount.
@eric, SL, etc.: As you all have agreed, the cutoff in the typical table of elements at 92 is just because of what's findable here on earth.
Supernovae, through the r-process, will probably produce elements right up to the relativistic limit (around Z=137 or so), but they'll decay long before the SN shock wave becomes transparent enough for us to see them.
Interestingly, there are trace amounts of (pre-1945) plutonium on Earth! The element has some isotopes with ~hundred-million-year half-lives. In the Oklo reactor, plutonium was produced through natural fission, and some parts per million still survive.
Oklo is one of those subjects I think is so cool that I love when people bring it up. And yeah, it counts as 'not man-made' production of Pu and Np and probably a bunch of other stuff. However, Oklo's natural reactors don't technically disprove the (strawman - nobody here is saying this) notion that starts don't produce elements higher than 92, because it wasn't a star.
Still, for anyone who hasn't read about Oklo's natural fission reactors, here you go. Sadly, the definitive 1976 Sci Am article about it is behind a paywall.
SL:
@eric
Don't blame me for astronomers discriminating certain heavy elements above 92 and leaving them out of the table :D
Pah, they're ALL metals when you get over Hydrogen.
Mind you, even being in the field, if the periphery, I've never really understood what the difference between a supernova and a hypernova is.
Nova and Supernova? Yes.
And even hurricanes and hypercanes (windspeed at the speed of sound).
But I had always thought that the difference between super and hyper novae was that there was going to be further fusion in the ejecta, so violent and dense the stellar atmosphere, even as it got blown off.
But the only things I find on readily available media is that it's just bigger.
I mean, bigger? Meh.
X rays and gamma rays overlap, the difference is where they come from.
"A hypercane is bigger than a supernova" seems irrelevant definition by circular logic.
My congresscritter tells me the claimed existence of transactinides is a liberal anti-Christian plot. He says no element is ever born that way, they're all just actinides that get together with other protons because they're sinful. And for some reason he wants to keep them out of public bathrooms.
:D :D :D
Shucks. I was hoping that green was due to chlorophyll