"We make the world we live in and shape our own environment." -Orison Swett Marden
If you had never heard of global warming before, how would you figure out whether it's real or not? And if it is real, how would you figure out what humanity's role in it is? To answer this, I've decided to do a three-part series on how you'd go about figuring this out, putting aside all politics, economics, opinion and any other non-scientific factors. If you missed part 1, you can check it out here; today we're going to build on that and talk about what determines the temperature of a planet with an atmosphere.
We last left off with Venus, the hottest planet in our Solar System. Despite being twice as far away from the Sun as Mercury, receiving only one-fourth the Solar output per-unit-area, and absorbing only about 10% of the Sun's energy (as opposed to 88% for Mercury), it's still hotter than Mercury by far. With an average temperature of 735 Kelvin (462 °C / 863 °F) regardless of whether it's day or night on Venus, this is entirely due to the Venusian atmosphere. The way it works is absolutely remarkable. It all starts with the Sun.
The Sun is, to use a tried-and-true metaphor, hot as hell. At least, that's true insofar as we can assume hell has a surface temperature of nearly 6,000 Kelvin! This radiation -- like pretty much all radiation -- has a very particular energy distribution known as (approximately) a blackbody distribution. (There's a little extra at very high wavelengths due to the effects of the Sun's atmosphere.) This ensures that the vast majority of the light that comes from the Sun peaks in the ultraviolet, visible, and infrared parts of the spectrum. That's what you'd get for pretty much anything you heated up to a temperature of 6,000 Kelvin: an energy spectrum that looks like this.
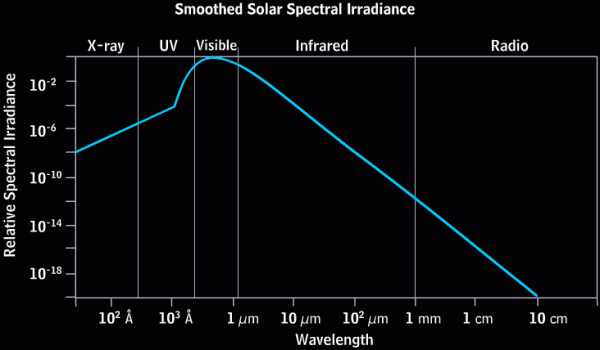
That's the energy that the planet's going to receive. In Venus' case, about 90% of that gets reflected back into space, and the other 10% gets absorbed. Now, here's the kicker: Venus -- and all planets -- then proceed to re-radiate that absorbed energy back into space! If Venus didn't have an atmosphere, like Mercury or our Moon, all of that energy would simply radiate away back into the Universe. Because Venus is at a lower temperature (like any planet), it radiates in the same general fashion that the Sun does, but the wavelengths it radiates at are shifted to much lower energies, lower frequencies, and longer wavelengths.
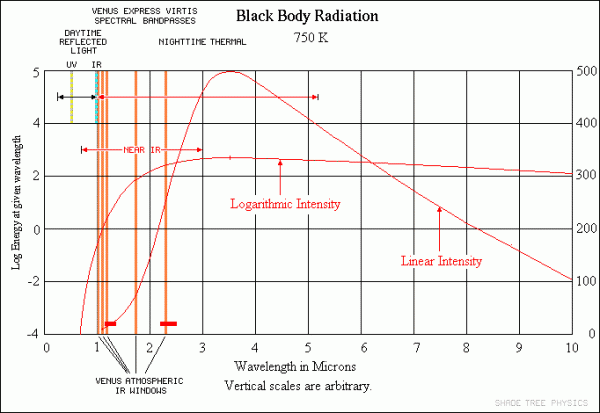
The "problem" is that many of the gases in Venus' atmosphere -- the gases that so easily let the Sun's light through -- are not transparent to the longer-wavelength radiation that Venus gives off! This is compounded not just by absorptive gas, but also by multiple layers of thick, absorptive clouds. So, what happens then, in terms of energy?
The Sun emits energy, Venus absorbs a portion of it, and then when it goes to re-radiate it into outer space, a large percentage of that energy gets absorbed by the atmosphere and re-radiated down to the surface. The surface then re-radiates the energy again, and once again, the atmosphere absorbs most of it, and re-radiates it down to the surface.
And this process continues. The thicker Venus' atmosphere -- and in particular, the thicker the atmospheric components that are opaque to the infrared light that Venus' surface re-radiates -- the longer that energy (in the form of heat) remains on the planet itself.
And that is why Venus is so hot!
These are the only photos (I know of) ever taken of a lander on the Venusian surface: the Venera 13 lander, which survived a whopping 127 minutes on the scorching 2nd planet from our Sun. (Its sister, Venera 14, survived for a respectable 57 minutes.) That's not bad, considering that Venus' surface is hot enough to turn metals like lead to liquid in a matter of seconds!
Now, back to Venus' atmosphere. It is incredibly thick: it contains about 100 times the number of molecules in Earth's atmosphere, and 96.5% of Venus' atmosphere is Carbon Dioxide. Most of the rest is Nitrogen, with trace amounts of some other molecules, including a little bit of the familiar Earth-favorite, H2O.
I highlight these two gases above all the others because they have significant absorption features in the infrared. Here's what the infrared absorption spectrum of Carbon Dioxide looks like:
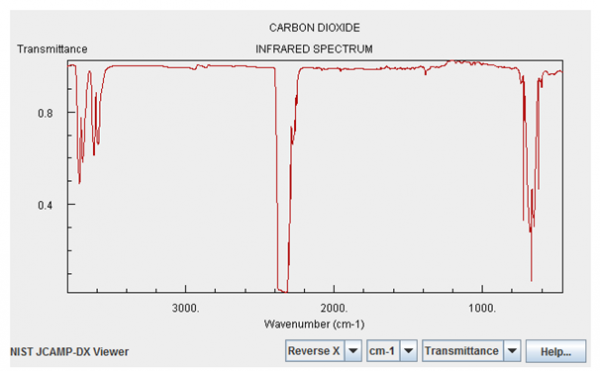
...and here's what it looks like for Water vapor.
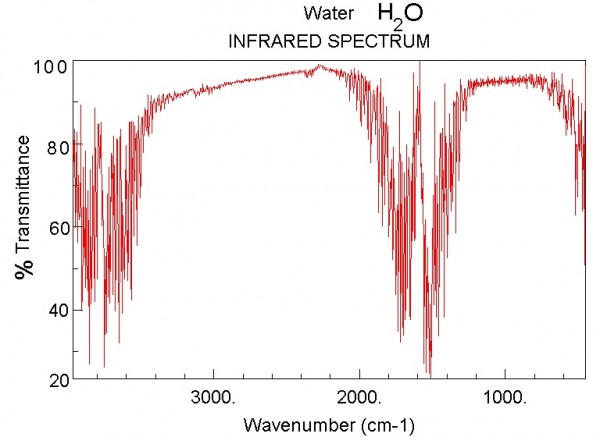
Now, the magnitudes shown here are not tailored for what the concentrations are on Venus. Water vapor is only about a quarter as important on Venus as it is on the graph above, but Carbon Dioxide is -- are you ready? -- about a quarter of a million (250,000) times stronger than what's shown. In other words, the Carbon Dioxide on Venus' atmosphere is primarily responsible for keeping Venus' heat from re-radiating back into space, and for trapping it for so long. Here's a quantitative look at what Venus' Carbon Dioxide does relative to the heat re-radiated from Venus' surface.
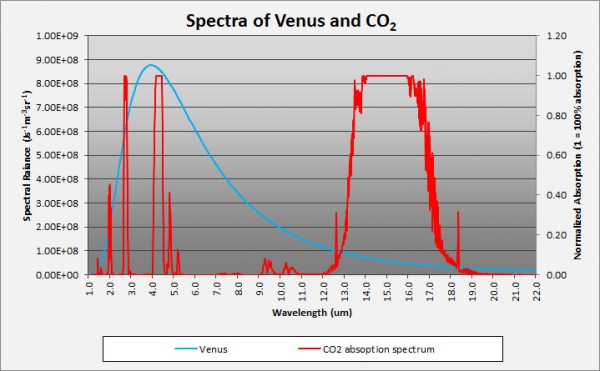
If Venus had no atmosphere at all -- if it were more like Mercury, just a sphere that absorbed most of the sunlight and then radiated it back into space -- its temperature would be about 340 Kelvin (67 °C / 153 °F), which is pretty hot, but nothing special.
The effect of Venus' atmosphere -- with all the clouds and gases in there -- is to act, metaphorically, like a thick, giant, insulating blanket; it keeps Venus warm via the same mechanism that blankets keep you warm: by absorbing its own heat and re-radiating it back on itself.
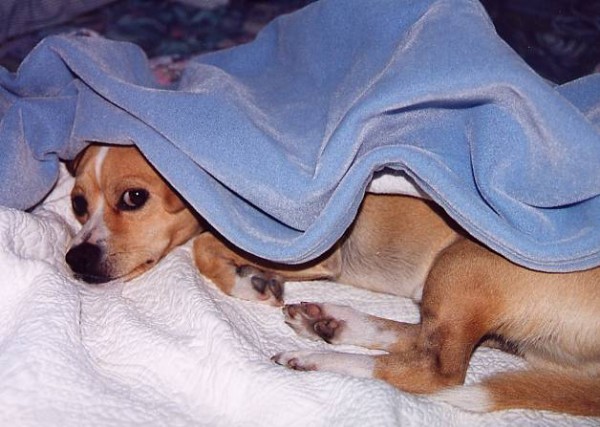
A heavier blanket will keep you warmer, and more blankets will increase the effect as well. It's not hard, with enough blankets, to heat yourself up to well above your normal body temperature; you have to be careful not to overdo it!
The Earth has a much thinner atmosphere, but it still manages to act like a blanket.
If it weren't for the Earth's atmosphere -- if our planet were more like the Moon or Mercury -- our planet's typical temperature would be 255 Kelvin (-18 °C / 0 °F), or well below freezing. We're not a frozen world, of course: the cloud cover, water vapor, methane and carbon dioxide, among other gases, keep our world about 33 °C (59 °F) warmer than it would be otherwise.
This effect was first discovered nearly two centuries ago by Joseph Fourier and worked out in detail by Svante Arrhenius in 1896. (Remember learning about acids and bases in high school chemistry? Yes, he's that Svante Arrhenius.)
All of it: water vapor, methane, carbon dioxide, every gas that absorbs infrared light, will act like a blanket. And when we add (or take away) more of those gases from our planet's atmosphere, it's like thickening (or thinning) the blanket that the planet wears. This, too, was worked out by Arrhenius over 100 years ago.
So that's what the Earth's atmosphere is: it is, depending on how you look at it, either a series of blankets, or a blanket of definitive thickness. You can add-or-remove blankets (or thicken-or-think your blanket) by adding or removing these various infrared-absorbing gases to the atmosphere.
And that's the idea that powers global warming, the greenhouse effect, and why planets with atmospheres are warmer overall than planets without them. Come back tomorrow, where we'll take a look at the final part of this series, and find out what's been happening on Earth recently, and see if we can untangle how to figure out whether global warming is real or not, and if so, whether it's been caused by human activity!
Update: you can now check out part 1, earlier, and the final installment, part 3!
- Log in to post comments
Coincidentally, this series of infrared photos showed up in my news feed today:
http://www.wired.com/wiredscience/2013/08/urban-heat-nyc/#slideid-223131
This is a nice summary with one important piece of the greenhouse effect omitted, which is: there is no atmospheric greenhouse effect unless the atmosphere has a temperature gradient. Because the earth's atmosphere has decreasing temperature with increasing altitude (well, the troposphere anyway), when radiation is absorbed at a higher altitude and hence lower temperature, that energy is subsequently radiated from a body at lower temperature and hence at a lower rate. You can think of the greenhouse effect as raising the effective altitude at which the earth radiates into space. The system warms until the outgoing radiation energy equals the incoming radiation energy. Here's a link to a decent explanation:
http://chriscolose.wordpress.com/2010/02/18/greenhouse-effect-revisited/
The greenhouse effect is described by the Schwarzschild equation (yes, it's the same dude).
Thank you very much. Looking forward to part 3.
Great post. I saw a denier blog post saying carbon dioxide is a "trace" element in the atmosphere, only 400 per million, and therefore couldn't be responsible for global warming. What would be a good way of explaining to a layman how the seemingly tiny amount of CO2 in the atmosphere is so consequential?
A trace amount of HCN in your bloodstream is fairly consequential, eh?
He is probably wrongly intuiting that the interaction of a CO2 molecule and a photon "uses up" the CO2 - so to absorb a lot of photons, you need a lot of CO2. That's not the case. In many chemical systems, a very small amount of a new reactant can cause a big change in the speed or end state of the reaction. The new reactant causes some reaction over and over again, any time it comes into contact with an unreacted compound (here; photon). Catalysis is a great example, though the CO2 absorption is not strictly speaking catalysis.
"which is: there is no atmospheric greenhouse effect unless the atmosphere has a temperature gradient"
I don't think this is strictly true. over at the Science of Doom he goes into some depth that you only need a CO2 molecule to absorb a photon, when it re-radiates, 50% can go up and 50% chance to go down...
Temperatures are measurements of a far greater number of molecules.. we can get a greenhouse effect without a temperature difference, although there is one.
It was a bit complicated and I didn't follow it all..
http://scienceofdoom.com/2010/07/17/the-amazing-case-of-back-radiation/
http://scienceofdoom.com/2012/07/23/how-the-greenhouse-effect-works-a-g…
There's a temperature gradient because there's gravity, Kevin.
However, that doesn't cause warming, which is why actual scientists working in the field use POTENTIAL temperature. I.e. the temperature that air would have if you adiabatically moved it to a standard reference pressure.
And in that case, apart from the effects of phase change in water (in the troposphere) and UV interception (stratopause), is seen in every gas part of a massive body that isn't generating heat itself (e.g. the Sun's interior).
Whether you cite the temperature gradient as relevant (in that you peg the temperature profile of the stable atmosphere as an adiabatic lapse rate from the stable Top Of Atmosphere radiative balance, 19C physics) or CO2's isotropic interception (showing the energy flows, 20C physics) is a matter of preference mostly.
Reality must accord a state where both views of the macro-scale fluid dynamics and the micro-scale radiative transfer come to the same result, since the latter is the reason for the former to hold.