“You do not become good by trying to be good, but by finding the goodness that is already within you, and allowing that goodness to emerge.” -Eckhart Tolle
It's been an amazing week here at Starts With A Bang, and you've been given plenty to think about. In particular, here's what the past seven days have seen:
- Heavy planets, light Sun? (for Ask Ethan),
- Revenge of the sparkles (for our Weekend Diversion),
- Genesis episode 6, the Moon (for Mini-Movie Monday),
- The slow dance that made you,
- Science by democracy doesn't work, and
- The camera that changed the Universe (for Throwback Thursday).
These were all fun to write, and as always, you weren't shy about sharing your opinions and the information you had. So which ones deserve a response? Let's dive in to your Comments of the Week!
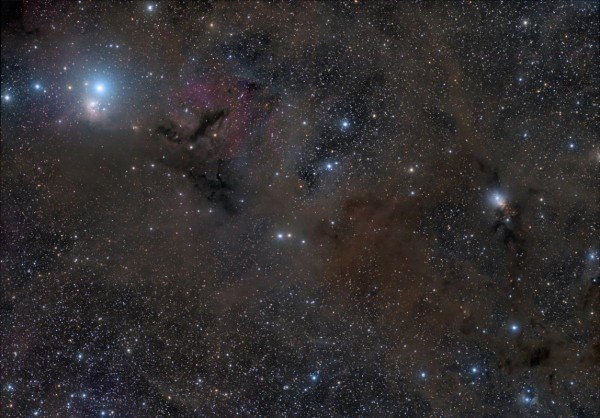
From david hurn on the first stars: "I presume the first stars had no rocky planets? How many generations did it take to achieve earth like planets?"
The first stars formed from the elements that were created from when the Universe was less than four minutes old. Some of those elements were radioactive and so they decayed, but all we were left with was about 92% hydrogen (by number), 8% helium, and less than 0.0000001% anything else. But once those stars formed, lived and died -- and the most massive ones go supernova in just a couple of million years, tops -- their "pollution" goes everywhere.
The pollution from the first stars comes in the form of elements such as carbon, oxygen, silicon, sulphur, iron, cobalt and nickel, among many others. Each supernova produces many thousands of Earth-masses worth of these elements, and because these clusters are small, pollution is widespread, quickly. While it might take a long time to get big rocky planets, or Earth-like rocky planets, or rocky planets that are quite rich in elements heavier than iron, we should start seeing the beginnings of rocky worlds in just the second generation.
The most likely place to find them? Gas giants -- Jupiter-like worlds -- that got too close to their parent star and had their hydrogen/helium envelopes boiled away.
Image credit: via http://shipyourenemiesglitter.com/.
From Pete A on glitter, glitter everywhere: "Van der Waals forces make me think of geckos, but after reading the article I shall also think of glitter — or perhaps a puzzled looking gecko wondering why it can’t remove glitter from its toes!"
So for those who don't know, Van der Waals forces take advantage of a unique property of atoms: that they have negatively charged electron clouds orbiting a positively charged nucleus. You might think of this as making it always "neutral", but the reality is that if you have any sort of electric field -- from polar molecules, externally, from adjacent electrical currents -- you wind up polarizing these atoms just a little bit. And if the electron clouds get offset from the nuclei at all, you can induce a weak, attractive force.
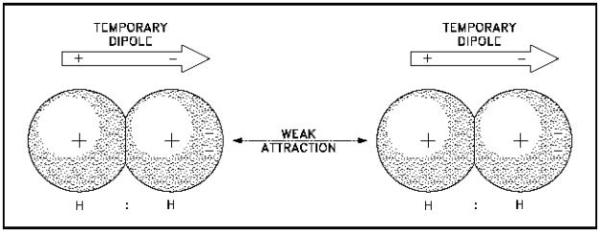
This is why geckos stick to anything (even teflon), why glitter gets everywhere and stays everywhere, and why Pete's dream animal -- a glittery gecko -- is a sticky-stuck mess. To those of you who don't like the words "sticky-stuck," here's a joke for you. Q: What's brown and sticky? A: A stick.
From PJ on our latest, Moon-filled mini-movie monday: "Like the presentations – keep them coming."
I'm not going to lie, PJ, these are a lot of fun to do. But they've also been a tremendous amount of work, for everyone involved but especially for me. We're probably going to go on hiatus for these, indefinitely, and I'll come out with a new Monday series (or miniseries) next week.
In the meantime, if you missed any of the six episodes, check them out below!
From Ragtag Media on the slow dance of the elements: "What about the collisions of neutron stars creating heavy elements, is that true or tbd?"
It's funny how a huge variety of elements there are, and yet how so many of them are made by different processes. "Normal" fusion produces helium from hydrogen, while the higher temperatures common to later times in a star's life cycle -- the red giant phase -- initiate helium fusion. This not only produces carbon, but can add various helium nuclei (two neutrons and two protons) to the elements, producing oxygen, neon, magnesium and other elements via that mechanism. In the most massive stars, carbon fusion occurs, then oxygen fusion, then silicon fusion and finally the iron/cobalt/nickel core collapses. A supernova, expelling heavy elements back into the Universe, is the result.
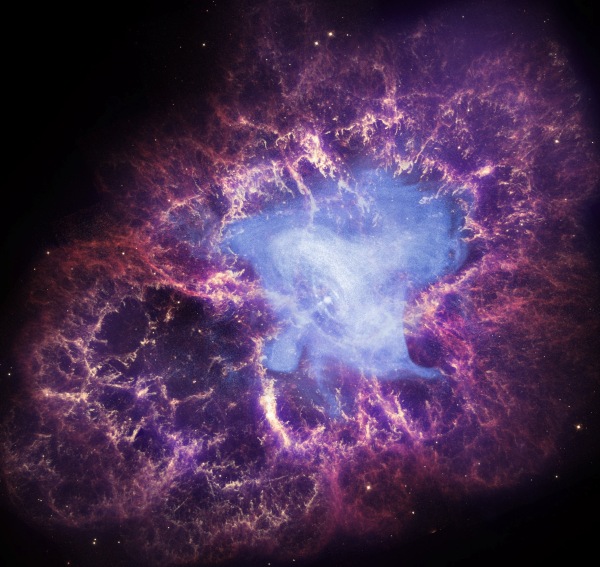
Oftentimes, at the center of this is a neutron star, clearly visible in purple in this composite X-ray / visible light / infrared image. Sure, the supernova expels a variety of elements into the Universe, but it's mostly those ones we mentioned earlier: oxygen, silicon, iron, cobalt, nickel, and also large amounts of aluminum, titanium and vanadium. To build up from those, we use the s-process in normal stars, which is what the slow romance I outlined earlier this week is all about.
But even though you can build all the way up to lead (and temporarily, bismuth) by the s-process, and even though you do produce heavy elements copiously in supernovae, the heaviest elements are most commonly produced by neutron star mergers.
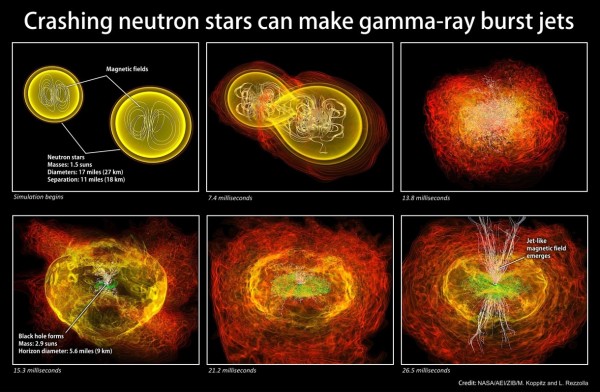
These are actually incredibly rare events -- happening only about once every 100,000 years in a galaxy like our own -- but each collision produces tens of thousands of Earth masses worth of elements that go back into the interstellar medium, with much of that being among the heaviest elements in the Universe. (I wrote an in-depth article about that process here.)
So when you see a table like this, below, of where the elements come from:
You should mentally add "neutron star mergers" wherever you see the "$" for supernovae. For pretty much all the trans-prometheum elements (since it's unstable), they may be more significantly produced by neutron star-neutron star mergers than by any other process, including both the slow-burning s-process and the rapid, supernova-based r-process.
Long answer, I know, but good comment!
Image credit: Edwin Hubble / Carnegie Observatories, via https://obs.carnegiescience.edu/PAST/m31var.
From Morgan on science, democracy and debates (and novae): "“Observations of Messier 31 (the Andromeda Galaxy) showed that there are many objects flaring up in that small region of the sky. They were similar in brightness to the novae that we see in our own Milky Way, except they were incredibly dim…”
I don’t follow; how can this be? By “similar in brightness”, do you mean they had a similar spectrum, or that the change in brightness followed a similar curve, or something like that?"
Here's the thing; there are two kinds of brightness when we talk about an object:
- Intrinsic brightness, which is all about the properties of an object that are objectively about the object itself, and
- Apparent brightness, which is how it appears to the human eye from our vantage point.
Intrinsically, these objects were the same as the novae we saw: same brightening time, same difference between initial brightness and peak brightness, same dimming time, same different between peak brightness and the dimmed brightness, same light curve, same spectral properties, etc. They were similar in intrinsic brightness.
But overall, they just appeared much fainter. Everything about them was the same as far as optical properties went, but they were just so much less bright in appearance, overall. Now, being farther away wasn't the only possible explanation for that: something -- like the dust found in a protostar environment -- could have been blocking the light in their environment, and that was one of Shapley's contention. (Helpful hint: if you ever want to sound smart asking a question in an astronomy talk, you can ask either about the evolution effects on a set of observations or the effects of dust/extinction.)
It turned out that Curtis was right, though, and that these objects appeared so dim because of the great distances to them.
And finally, from Major Tom on Hubble, its camera(s) and its great images: "Note how deeply dark space is – you can’t see the stars! Nor the sun, you can only see light that has reflected off of something."
Although you are seeing the dark depths of space, it isn't true that you can't see the stars. For one, even in an image like this, there are stars in our galaxy that are visible. You can see them wherever the diffraction spikes are: I'll point them out for you.
But more than that, the individual galaxies you see are made of stars, and their light comes to us one-photon-at-a-time, added up over hours and hours, days and days. Zooming in on just a tiny region of this image (in the lower right), what you're seeing is the sum of the light from billions of stars. Only, it's billions of stars after traveling a distance of billions of light years!
The only "reflection" is off of Hubble's mirror and into its cameras. And -- in this case -- it was the amazing WFPC2 camera that was sensitive to single photons that changed our view of the Universe forever.
Thanks for a great week, everyone, and I hope you enjoyed your weekly comment roundup!
- Log in to post comments
Ethan, Thanks for the Videos, They are a great adaptation. and add a relative value. Thanks for the extra work. It;s appreciated, Even if I am a Bible thumpin God Fearing Believer I Still have a desire to exploe new things
Remember, The Earliest S chools of Education In America Only Had thier Bible to Study and did NOT shun higher fields of thought.
Anyhow.
Thanks Ethan
Your The Best