"If you're a reporter, the easiest thing in the world is to get a story. The hardest thing is to verify. The old sins were about getting something wrong, that was a cardinal sin. The new sin is to be boring." -David Halberstam
It was only a few months ago that both collaborations at the Large Hadron Collider in CERN -- CMS and ATLAS -- announced the discovery of a new particle at about 125-126 GeV of energy: something that looked an awful lot like what the Standard Model predicted the Higgs Boson should be.
This was the result of decades of investing, thousands of people's tireless efforts, and unprecedented international collaboration. But in many ways, this was also only the beginning. Yes, by combining all of the data, we were able to tell that there was a new particle there of a certain mass, that it's a boson (as opposed to a fermion), and that it has many properties consistent with a Higgs Boson.
But there were also some important questions that needed to be asked, that we weren't ready to answer yet.
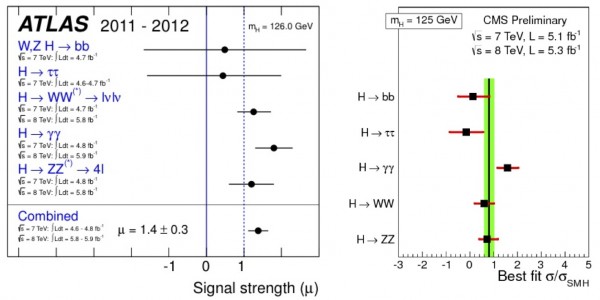
1.) Does this particle, in fact, behave and decay the way the Standard Model's lone Higgs Boson is expected to?
When the discovery was first announced, only a few decay channels had been measured. A number of them were suspiciously silent -- although that could have been just due to low statistics -- while one of them (the two-photon channel) had almost triple the expected signal. This could have meant that this particle wasn't the Standard Model Higgs, but was instead a different type of particle. This is akin to if you flipped a coin ten times, and it came up heads eight times, you might start to worry that your coin was biased against tails, or unfair in some way.
You've got to remember that over at the LHC, they're colliding millions of particles together every second, in bunches just nanoseconds apart. You've got to very quickly sift through your data and throw away the 99.999% of it that's uninteresting (i.e., where no rare particles are created) and then record-and-analyze everything that you kept. The Standard Model, regardless of the Higgs, produces a mountain's worth of data. (Literally; if you wrote all the data that the LHC hasn't thrown away to CD-R and stacked it, you'd have hit the International Space Station by now.) And you've got to find -- among that mountain -- the few rare gems where a Higgs boson, for just 10-25 seconds, was created.
And now the data's come in. And what we found is that those rarer decay channels are seeing decay rates consistent with Standard Model predictions, and that the two-photon channel is now much closer to what's predicted. In other words, now that we've flipped the coin 100 times, the fact that we have 58 heads doesn't seem so remarkable anymore.
So score one for "Standard Model Higgs."
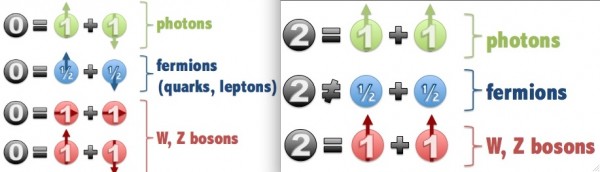
2.) What is the spin of this particle?
All particles have an intrinsic amount of angular momentum, which physicists refer to as spin. There are fermions, which have a spin in half-integer increments (e.g., ±1/2, ±3/2, ±5/2, etc.) and bosons, which have spins in integer increments (e.g., 0, ±1, ±2, etc.). Because photons are spin-1 and we knew the particle the LHC found could decay into two of those, we knew that it was therefore either spin-0 (because 1-1=0) or spin-2 (because 1+1=2), but couldn't be either a fermion (because there's no real way to combine 1 with 1 to get a half-integer) or a spin-1 boson (or higher).
But there are certain, rare decays that would tell the difference. For example, a spin-o boson could decay into two τ-particles (which are spin-1/2), because (1/2-1/2=0), but a spin-2 boson couldn't. More subtly, there are some sophisticated differences in distribution that will show up in the two-photon channel and the four-lepton channels if the progenitor particle was spin-o or spin-2. The new data show both evidence that this new particle decays into two leptons -- consistent with spin-0 -- and also that the two-photon and four-lepton channels favor spin-0 over spin-2.
So, score two for "Standard Model Higgs." And finally...
3.) What is the parity of this particle?
This is perhaps the most challenging of all. Parity is a scientist's way of asking the world if the laws it obeys are the same as a mirror's reflection, like the letter "O", or flipped when you reflect it in a mirror, like the letter "E".
For the Higgs boson, it's supposed to behave like the letter "O", which means it should have positive parity, as opposed to the "E", which would be negative parity. This is too difficult to test by directly measuring the Higgs boson, as it doesn't live long enough. But what we can do is look at the decay products; if there are four (or more) particles that wind up coming out, by measuring the angles the four particles come off at relative to one another, we can figure out whether it's positive parity (like the letter "X") or negative (like the letter "K").
Well, the data has come in, and pretty definitively, ATLAS has concluded that this new particle has positive parity, just like the Standard Model's Higgs Boson should.
And so that makes the Standard Model Higgs three-for-three on the prediction front. So finally...
4.) What does this mean for the possibility of extra particles beyond the Standard Model?
As you likely well know, there's been a lot of excitement over the possibility that yes, there's the Higgs Boson, but that there could be many more particles that the LHC will discover! For instance, if Supersymmetry is correct, there should be superparticles (partner particles to each of the Standard Model ones), as well as multiple Higgs Bosons.
So far, no evidence of these has shown up. But what about hints? Those of you who've been keeping your ear to the rumor mill may have heard Joe Lykken say the following:
"If you use all the physics that we know now and you do what you think is a straightforward calculation, it's bad news... [t]his calculation tells you that many tens of billions of years from now, there'll be a catastrophe... [i]t may be that the universe we live in is inherently unstable and at some point billions of years from now it's all going to get wiped out."
What Lykken is referring to is that, if you know how all the particles of the Standard Model interact, and you know the masses of all of those particles, you can determine whether the Universe is stable all the way up to arbitrary energies, or whether there's an inherent instability, and the Universe is only quasi-stable, up to some finite energy. If there's an instability, that means that either the Universe is in a quasi-stable state that it won't stay in forever, or there are new particles before we reach that cutoff energy.
There are three Standard Model particles whose masses still have a fair bit of uncertainty: the Higgs, the top quark, and the W-boson. Accepting them at their best-measured values right now means that the Universe is stable up to about 1011 GeV, or a factor of 10 million higher than the LHC can reach. I don't know what "straightforward" calculation Lykken is referring to, but the calculations I've seen indicate the Universe will remain quasi-stable not for tens-of-billions of years, but quadrillions, at least, even if there are no new particles.
But if we include the present uncertainties in those particle masses, and accept that maybe the top quark is off by about 1.7% than the accepted best-measurement right now (less than a 2-σ uncertainty away from the central value), then the Universe is stable up to arbitrarily high energies, and will remain stable, forever. With no new particles. So while what Lykken said -- with some caveats -- is definitely within the realm of possibility, there's no experimental or observational evidence to indicate that it's at all likely. The Standard Model is what we've got, and until the experiments show otherwise, there's no reason to believe that, other than something to explain neutrino masses (and possibly a lack of strong-CP violation), there's anything else out there.
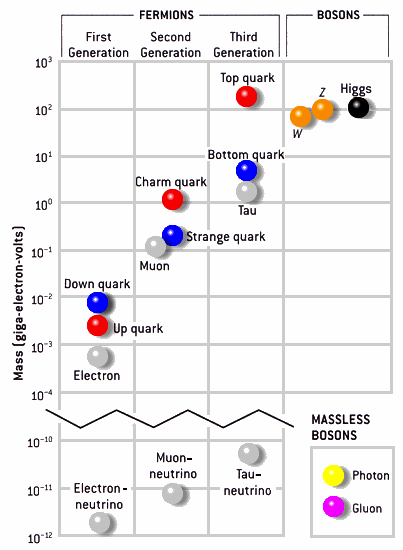
Of course, the LHC is undergoing an upgrade, and will resume the search for new, exotic particles at even higher energies in a couple of years! But for right now, if we're following the data, the smart money is that the Standard Model is right, this is the boring Standard Model's Higgs Boson, and it would be a huge surprise if the LHC found anything else. But we've been surprised before, and that's why we look!
And for those of you who missed it last night, I had the opportunity to tell the world -- on Live TV -- about the new findings!
- Log in to post comments
That Odd Parity diagram looks like a fun time!
So if the Higgs is just a Higgs and asymptotic safety holds, what specific theories bite the dust? You mentioned multiverse, technicolor and super symmetry in your earlier post about asymptotic safety. Is string theory included? Or do they just make claims that are so far from testable that facts can't touch them. Any further thoughts on the soundness of the asymptotic symmetry math? I've waded through 1.4 technical papers on it and saying I get the gist of the math is probably an insult to gists. I think it's fascinating but theorists seem to think asymptotic safety is not something they want to explore (could it be job insecurity?). Even if I don't get the math, the philosophical and theological implications are fun to mess around with. bc
Hi.
Again, very nice post!
This may be a stupid question, but what does "unstable" mean in terms of the universe?
You say energies a million times higher than the LHC has...what would happen if the LHC would run at these energies?
Many thanks.
Saw this picture on Facebook, had to share!
http://fbcdn-sphotos-c-a.akamaihd.net/hphotos-ak-ash3/578549_4875835612…
(You're not a dick BTW)
Last I read is that Carlo Rubbia is proposing building a muon-muon colider in order to study Higgs in detail.
Mick:
A similar comic from SMBC:
http://www.smbc-comics.com/index.php?db=comics&id=1777#comic
I think that is exactly the wrong take on it. Why is the SM so finetuned and why does it come out as bordering on instability?
If you are looking for new physics instead of trying to save the old, that is a question to take seriously. Not hoping, likely then against hope, that there is a large enough measurement error to make any interesting physics go away.
Sure, it could happen. But I don't think that it is the "smart" money, given the current context. (As described here of course, there are much detail laymen doesn't know or wouldn't get anyway. Even so, if it is detail instead of the large considerations that "saves" old physics from the brink of finetuning, it would be interesting.)
Oops, wait. That paper and its linked article wasn't about the usual calc's on vacuum quasistability. Asymptotical safety, huh? Definitely fitting the bill of "interesting detail", have to read about it.
Still, it would only happen if you don't account for dark matter, doesn't it? "No new physics until Planck scales." So I don't get the thinking behind accepting it, we do see dark matter.
@Bruce: No, multiverses wasn't damned. Besides DM and neutrino oscillations (and strong CP et cetera), dark energy would remain to predict without new particles to help.
You could even say that asymptotic safety leaves to anthropic selection à la Weinberg to account for dark energy (and hence multiverses), which would be an interesting take considering earlier articles here.
@T Larsson: To quote Ethan (Oct 17, 2012)-- " a Higgs Boson with a mass of 126 GeV, with a very small uncertainty (±1 or 2 GeV), would be damning evidence against supersymmetry, extra dimensions, technicolor, or any other theory that incorporates any new particles that could be found by any accelerator that could be built within our Solar System." I have confessed my ignorance about the math and I gladly admit that I substituted "multiverses" for "extra dimension".
That being said, Shaposhnikov and Wetterrich's estimation of the energy level that the Higgs would be found at was rather impressive (at least to someone like me who only grazes through blogs like this one, for amusement and a bit of horizon expansion) and if multiverses do not involve extra dimensions, I would appreciate a concise explanation of what the distinction between the two is. Or some hint of where to look for more information that would shed more light on your point.
The question remains: If the Higgs is just a single Higgs and the math underlying asymptotic safety is sound, then what are the implications for the various theoretical postulates for the basis of matter and the basic forces? Ethan's organizational chart is nice but lacks a little on the explanation side. But then saying specifically that someone's pet theory of reality is probably unlikely, may not be the way to win friends. bc
Torbjörn Larsson, keep in mind he's just talking about new particle physics discoveries. There are of course other physics mysteries to be explained -- maybe even a deeper understanding of the SM that explains the fine-tuning. But nothing we will discover with a particle accelerator.
Maybe.
But that's what the current evidence suggests.
great post! you were great on TV.. can you explain what image "Image credit: CMS group / Imperial College London; minor edits by me." is saying?
I see the line at 125 GEV, and I see Zy and yy nearby.. and I guess I know that bb' tt' and WW, ZZ are bottom and top quark decays, and W0 and Z(+,-?) particle decay channels..
but how the heck do you read that thing?
Kevin,
It's just a graph that described what the standard-model-predicted branching ratios of the Higgs should be.
In other words, if we make a Higgs boson of 125-6 GeV, it should decay to a Z (the neutral one) and a photon about 0.1% of the time, to two photons about 0.2% of the time, to two Z's about 1.2% of the time, to charm-anticharm about 3.5% of the time, to two gluons and to tau-antitau about 7% of the time apiece, to a W+/W- pair about 11% of the time, and to bottom-antibottom about 70% of the time.
That's the standard model's prediction for a Higgs of the observed mass. The next step is to see -- once we get the error bars down with more statistics at the higher (post-upgrade) energy -- whether, in the graph below that one, whether the experiments line up with the Standard Model prediction, which is the dotted line of μ=+1.
ok sorry.. not getting it.. what do you call such a plot? I can look it up. so, the left scale is BR(H) is the Higgs branching buckets and the bottom is .. the mass of the particle when detected?
but then we would not have tracks because the weight of the particles is always the same..? "W+/W- pair about 11% of the time, and to bottom-antibottom about 70% of the time."
- but they are both charted at the top of the plot... I would think the bb' would be all alone up top and the other spread out beneath??
so as a for instance.. look at where tt', gg and WW intersect at the 125 GeV line. what is that telling me?
thx in advance!
kd
Just scrolling slowly and that picture of the dude for Odd Parity/Even Parity made me look twice.
The second one as I scrolled down looked like the same dude but doing the can-can and SOMETHING was dropping from the "lower bifurcation".
BRAIN BLEACH! STAT!!!