"There are no physicists in the hottest parts of hell, because the existence of a 'hottest part' implies a temperature difference, and any marginally competent physicist would immediately use this to run a heat engine and make some other part of hell comfortably cool." -Richard Davisson
The Large Hadron Collider is the most powerful particle accelerator in the world, capable of accelerating protons absurdly close to the speed of light: 99.9999991% of the way there! Within a 26-kilometer-long ring, largest in the world, protons are accelerated both clockwise and counterclockwise up to these incredible speeds, and smashed head-on into one another.
But these collisions don't take place willy-nilly inside the ring. Instead, the protons are consolidated into tiny bunches, and strategically crossed at only two specific collision points: deep inside the two tremendous detectors designed to collect and analyze the debris from proton-proton collisions. These two detectors are ATLAS, which stands for A Toroidal LHC ApparatuS, and is huge!
ATLAS, at 44 meters (144 feet) long by 25 meters (82 feet) in diameter, is the largest detector ever built for an accelerator-collider, and weighs in at 7,000 tonnes. The other one is CMS, the Compact Muon Solenoid, and is the second largest detector ever built for these purposes. Although it's "compact" in size only in comparison to ATLAS, it outweighs it by nearly a factor of two, coming in at 12,500 tonnes.
The two proton beams circle clockwise and counterclockwise -- one atop the other -- inside this 26 kilometer-long tunnel. Traveling in bunches spaced only 25 nanoseconds apart, they become highly focused and directed at one another, allowed to cross one another only inside each of these detectors.
Inside, a tiny fraction of the protons will collide with the ones going in the opposite direction, smashing the protons apart and producing highly relativistic debris. But these protons are moving incredibly fast, at 299,792,447 m/s (just 11 m/s slower than lightspeed), and traveling in bunches of literally trillions of particles.
These detectors face an amazingly difficult, twofold task: sifting through a firehose of collision data (an estimated 600 million collisions-per-second), while simultaneously detecting exactly which of the debris particles came from which collision (in which direction, with which energy) and from where. This is no small task when your raw "results" look like this.
But after sifting through (literally) hundreds of trillions of these ultra-high energy collisions, the ATLAS and CMS detector teams have released their preliminary results on the search for the Higgs boson, flooding the news media.
Before we plunge in to the results, like Tommaso, Clara and Peter have (admirably) done, let's take a look at what we're attempting to find, so that we won't get swamped by speculations and misinterpretations.
These are the particles in the Standard Model of elementary particles. Quarks, coming in six flavors and three colors, leptons, of the charged (electron-like) and uncharged (neutrino-like) variety, as well as the force-carrying particles: the photon, the eight gluons, and the very heavy weak bosons (responsible for radioactive decay), the W± and the Z0. All told, these particles and the way they interplay with one another fundamentally and successfully explains every phenomenon ever observed, with the sole exception of gravitation.
Each of the quarks and leptons has an antiparticle, too, and over the years -- mostly as a result of particle accelerators and colliders -- we've actually detected and discovered all of them. All of them, that is, except the elusive Higgs boson, whose role is to give rest masses to all the other particles. And it's no wonder we haven't discovered it yet: it not only takes an incredible amount of energy to produce a Higgs, but the only theoretical processes that can do so even a fraction of the time are themselves incredibly rare!
Discovering the Higgs -- and where (i.e., at what mass) we discover it -- is incredibly important for telling us whether the Standard Model is likely the entire story, or whether there needs to be physics beyond it to explain what we see. For example, if we find the Higgs at energies of less than 120 GeV (where the mass of a proton is just under 1 GeV), there's very likely supersymmetry in nature, a speculative but theoretically interesting idea, that would double the number of fundamental particles.
If, on the other hand, there's no Higgs at all, anywhere then the Standard Model must necessarily need correcting, and the best idea we have is that quarks are actually not fundamental, but composite particles that can be broken apart, in a theory called Technicolor. Technicolor, of course, has its own technical predictions, and so if it exists, the LHC should see evidence of that, instead.
Of course, the Standard Model could also be right, and could also be the full story. What would it look like if there were a Standard Model Higgs and nothing else?
We'd expect that the Higgs would come in with an energy (convertible to a mass via E = mc2) of somewhere between 120 GeV and 140 GeV. But mass, in this context, doesn't mean what you would expect. And that's Heisenberg's fault.
You almost certainly think about mass -- which is equivalent to energy -- as a fixed, constant property of an object. That if you make a million of a certain type of particle, they'll all have the same exact mass. And this is true, kind of.
It's exactly true if your particle is stable, and lives an arbitrarily long amount of time. But if your particle lives a short amount of time (Δt), the inherent uncertainty in its energy (ΔE) becomes large, thanks to Heisenberg's uncertainty principle. For a long-lived particle, the uncertainty in energy (and hence in mass) is negligible. But for our purposes, what this means, theoretically, is that a very short-lived particle actually picks up an intrinsic uncertainty in its mass.
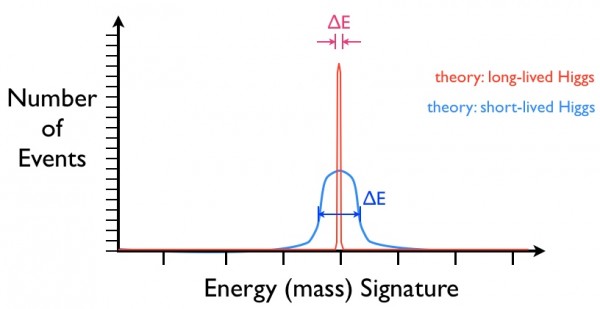
So if you make a bunch of them, they're not all going be at exactly the same energy; they're going to appear spread-out over a range of energies. What's even worse about the experimental search for the Higgs is that, even if you produce a few of them, and even if your detector performs its herculean task perfectly, it's only going to measure a finite number of events with a finite resolution. So the best you can hope for, with a realistic model of the Higgs, is for an experimental signature that looks like this.
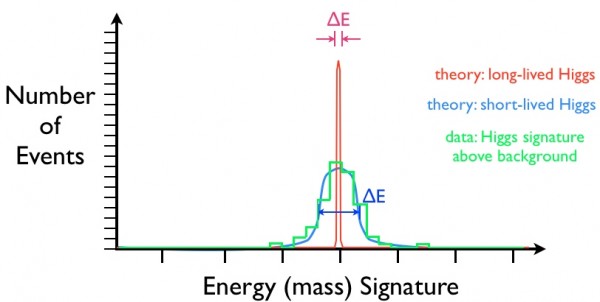
And finally, just as there are multiple pathways for making a Higgs, there are multiple pathways for the Higgs to decay, which it will always do in incredibly (like, yoctosecond-scale) short order. So if you want to find the Higgs, you need to look for its decays into all sorts of things: photons, W-bosons, quark-antiquark pairs, gluons, leptons, etc.
Based on the number of Higgs particles you think you should be producing, how many of these events can you reconstruct into supposed Higgs? That is, you're also going to produce photons, W-bosons, quark-antiquark pairs, gluons, leptons, etc., whether there's a Higgs or not. So you calculate your expected background without a Higgs, do your experiment and measure what you actually see, and then ask yourself: do I see something interesting, and if so, how likely is it that it's real, rather than just a statistical fluke.
Because we've "fluked" ourselves good once before.
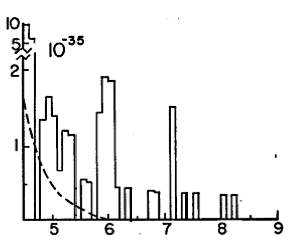
Back in 1976, there were only four quarks that had been discovered, but suspicions were incredibly strong that there were actually six. (There are, in fact, six.) If you look at the above graph, the dotted line represents the expected background, while the solid line represents the signal published here from a E288 Collaboration's famous Fermilab experiment. Looking at it, you would very likely suspect that you're seeing a new particle right at that 6.0 GeV peak, where there ought to be no background. Statistically, you can analyze the data yourself and find that you'd be 98% likely to have found a new particle, rather than have a fluke. In fact, the particle was named (the Upsilon), but when they looked to confirm its existence... nothing!
In other words, it was a statistical fluke, now known as the Oops-Leon (after Leon Lederman, one of the collaboration's leaders). The real Upsilon was found the next year, and you shouldn't feel too bad for Leon; he was awarded the Nobel Prize in 1988.
But the lesson was learned. It takes a 99.99995% certainty in order to call something a discovery these days. So with all of this in mind, what did the CMS and ATLAS collaborations find, and did they discover anything?
Above is the results from the CMS team's search for the Higgs in the high mass range: up to about 600 GeV. What you're looking at shows a dotted line from what the "expected background" is supposed to be. Anything that falls within the green range is just where it's supposed to be, anything that falls within the yellow range is perhaps suggestive of something interesting, but not at all compelling. What we're really hoping for is whether anything rises up and above that yellow region (into the white), which could be construed as evidence (if not yet a discovery) of something new and interesting. Barring that, anything that sinks down below the red line -- Biggest Loser-style -- is ruled out as being the Higgs.
As you can see, CMS sees nothing in the high-mass range that's at all interesting. Well, what about in the low-mass range? Remember, that's the one we expect to be more interesting!
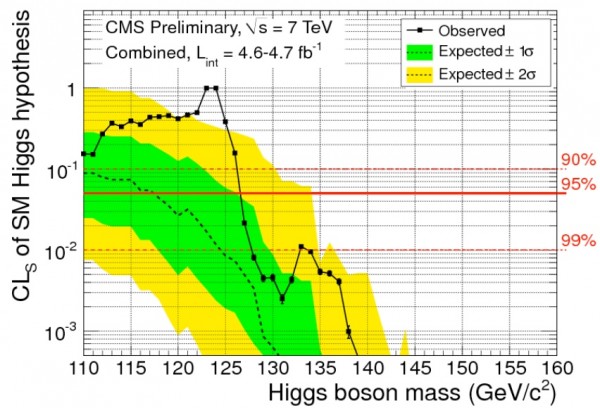
Ahh! Now, this is not a discovery, and it's not even particularly strong evidence, but if I were a detective, I would have just uncovered a set of fingerprints looking at this!
First off, the interesting part of the "low-mass" range just got a lot smaller; anything for the Higgs of over about 127 GeV is looking pretty unlikely, thanks to CMS. But looking just under 127 GeV, there is an excess. It isn't a huge excess, but there's definitely something interesting there. The next step would be to look at the different decay channels. Because if this is evidence of a Higgs, I should see an excess in each of the channels, and of roughly the same magnitude. What do we get when we break it down?
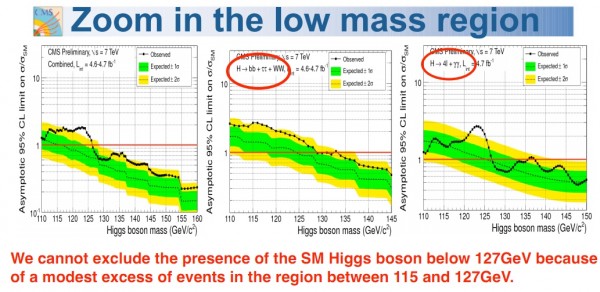
This is so hard. I look at this and I know, immediately, that I need more data to know whether there's something interesting, or whether I'm just seeing a fluctuation. If all I had was the CMS data, I'd be well within my rights to publicize what we've ruled out, and to speculate that if there was anything interesting, it would be in the low-mass range.
But we are not restricted to CMS results, there's a whole other collaboration doing these experiments! So let's find out: what did ATLAS see?
Taking a look at the high-mass range, it's almost the same story as CMS. Heavy exclusions, mostly, with hardly anything appearing even mildly interesting. (Maybe that little bump at ~250 GeV, but even that's still in the yellow.)
But -- here's the deal -- based on what I've already seen from CMS, I'd be really interested if ATLAS, working completely independently, saw something in that same below 127 GeV energy range. So what do they see at low energies?
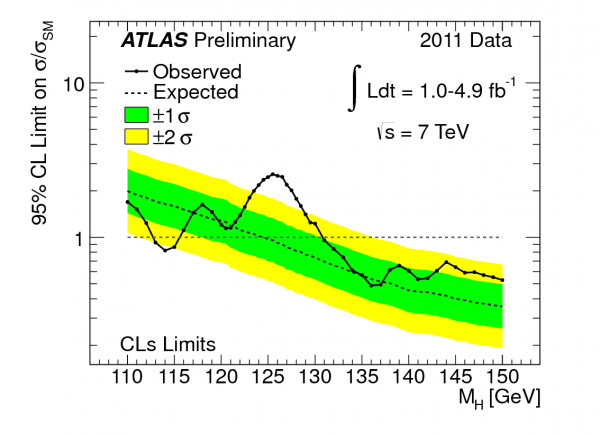
You're kidding, right?
Right there, at 126 GeV, is a bump in ATLAS's data?! As others note, this is really interesting. Officially, the significance and strength of this signal -- if there is a Higgs right at 126 GeV -- is perfectly consistent with a Standard Model Higgs boson, and nothing more. Furthermore, independently of CMS, anything over 131 GeV is ruled out, all the way up to that bump at ~250 GeV.
But, as you may have guessed, this is not significant enough to claim a discovery. It's estimated that the Large Hadron Collider will need to take about 3-4 times as much data in order to know at the desired confidence levels whether or not there's a Higgs at the low-end of our mass range. Although it's extremely suggestive that both CMS and ATLAS see something there, the evidence isn't nearly as strong as we'd like. That said, if you combine all the data from the different ATLAS channels, what do you get?
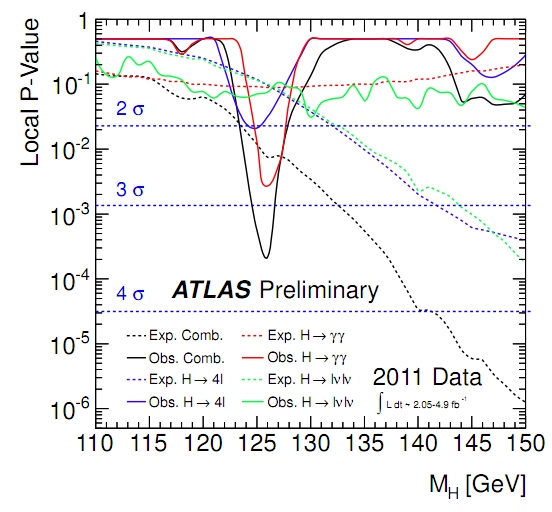
The odds that you'd get a combined fluctuation like this are something like 1-in-50, if you consider the entire range of where we looked. However, if you restrict yourself to looking in the same place that CMS saw their fluctuation, the odds of that happening are now something appallingly small, like 1-in-2000. It still isn't good enough to claim discovery, but it's striking enough that if you asked me to bet, I'd bet you even money that they confirm a Higgs somewhere around 126 GeV by the end of 2013. And that's what I'm hoping for, too.
Some of the best physics takes on this that I haven't yet linked to can be found here, here and here, and if you need a stream of constant, up-to-the-minute LHC news, I've got you covered. So now, you know what's been found at the LHC and what it means for the Higgs; there's nothing left to do but enjoy the findings, wait, and hope! Maybe I'll see you back on the news sometime soon!
- Log in to post comments
Three questions:
1) When the confirm the mass of the Higgs (at say 126 GeV), what does that tell us about the rest of the Standard Model? That is, does the weight of the Higgs help fix any other constants, or tells us the value of constants?
2) More basic question: If the Higgs field gives everything its rest-mass, why is it so impossible to make a Higgs particle? What's going on here?
3) If we find the Higgs by the end of 2012 or 2013, that's a stupendous achievement, but what else is the LHC going to be good for after that?
Looking at the ATLAS results, why the spike at 250, and the dip at 375? Do they indicate anything?
Great article, as always. Puts the search and the finding within grasp of understanding. Circulated widely.
Small, unsolicited piece of feedback: I'm not a fan of the use of TrapIt as links to other concepts and stories. For one, I like to mouseover on the URL to see where it's going to take me and whether I'm likely to want to click it. Second, I just don't particularly like the TrapIt interface. Could be just me though.
Also, minor typographical error note: What you're looking ((at)) shows a dotted line from what the "expected background" is supposed to be.
I guess the instability of the Higgs has me stumped too, how can it be the one part giving everything mass if it doesn't stay around?
Tantalising....
It would be a very frustrating coincidence if that is what it is.
Here's to more data :)
The fact that there is a spike at 2x126 GeV and a dip at 3x126 GeV is tantalizing as well. It suggests either some property of the Higgs Boson or some shared mechanical flaw in the detectors at those frequencies.
Best explanation of this process that I've seen yet. Thanks Ethan!
Okay, it's official!
Tonight, 7 PM (Pacific Standard Time), come see me live on KGW's News @ 7 in The Square: http://www.kgw.com/thesquare/about/Live--7-Boothcam--Chat-Room-10417500…
Can't catch it live? Get the video recap here after the show: http://www.kgw.com/thesquare?more=y
At 99.9999991% of the speed of light, what is the mass of a proton?
may i suggest shaving your facial hair, you look like an evil, scary, maniacal freak, or maybe you actually are!!!
may i suggest shaving your facial hair, you look like an evil, scary, maniacal freak, or maybe you actually are!!!
^^hahahahaha. Great physics amazingly well explained and what is the detractors reply?...
Re Candiru @9;
Using the gamma equation (if'n I did it right) with that speed gives the mass roughly 7,500 times rest mass.
Hi Ethan,
Thanks, a nice piece as being very informative having me now leaning towards thinking theyâve hit pay dirt this time. The thing I would like to know is whatâs planned now to find out if this good bet is a sure thing or nature simply playing tricks. I seem to recall some place reading that the energy range now being explored is fraught with problems as being one difficult to distinguish signal from noise.
Best,
Phil
Ethan - these trap-it links never present the actual link. Now I have no idea whether you linked to this one
http://www.science20.com/alpha_meme/higgs_discovery_rehabilitating_desp…
or this one:
http://www.science20.com/alpha_meme/light_higgs_discovered_and_about_de…
;-)
Please use the usual linking - or am I the only one having this problem (because I am in China or something like that)?
Hi Ethan,
Great explanation! May I ask what determines the standard deviation in these experiments? Also any clue what the valley at ~380 GeV in the Atlas detector is?
It would be wonderful to hear you give us insight into what ATLAS and CMS are actually doing. I expect it's pretty complicated stuff, but I'm sure you can explain it to us. Thanks for the great stuff!
"2) More basic question: If the Higgs field gives everything its rest-mass, why is it so impossible to make a Higgs particle?"
Because we need to "boil it out" of the structure of the universe, where it exists as a virtual particle. We need to give it energy to free it, to make it appear real to our inspection.
And being very heavy, that requires a lot of energy to do.
Analogy time: you can't see the air, but you can feel its effects. You need to do a lot of work (magnify) to make the constituents visible.
"but what else is the LHC going to be good for after that?"
What use is a bomb you're never going to use?
What use is a Higgs Boson discovery in 2012? But you need an LHC to find one in 2012. After that, it's now more useful than a spent rifle cartridge (even if not by much), but that doesn't make the making of the round useless in the first place.
Wow:
Hey, I'm not cracking on building the thing. Finding the Higgs will be a wonderful and important accomplishment. But after that, I figured that physicists might not know what to do with a device that can go up to 14 TeV when it found the Higgs at a tenth of that.
Apparently there's some stuff with supersymmetry that they can go looking for, but it still seems to be more an arduous ruling-out process than anything else. Nice blog post on what's next over on Cosmic Variance:
http://blogs.discovermagazine.com/cosmicvariance/2011/12/13/first-glimp…
You did a fantastic job explaining all this to an ordinary person. Thank you.
Implosion
Exhausted the universe falters
strains against its tether energy spent
journeyâs end triggers return
the cosmos shudders glows a dull red
pauses before yielding its mandate
gravity tightens its grip
the universe recoils design shatters
collapse starts slowly cause and effect invert
time runs backwards the past now future
the implosion feeds on itself
consumes the oldest star clusters
swallows super giants and white dwarfs
gorges on mighty pulsars and nebulae
rips away planetary atmospheres
gulps down massive quasars devours galaxies
inhales neutrino-rich darkness
black holes converge explode vaporize
white-hot plasma ravages the void
a cosmic furnace a trillion miles across
energy densely packed heat at billions of degrees
three minutes atomic nuclei disintegrate
the universe a shambles a raging vortex
10 billion miles in radius at terminal velocity
gravity at infinite power seconds remain
the ferocious inrush obliterates space and time
a billion billion years now a microsecond
the fireball claims the last particles
matter flames out of existence
the ravenous sphere spins down
a billionth of a second from its genesis
quiescent singularity
opaque silence
requiem
history is extinguished
time and space never were
the universe is gone
save for a subatomic speck
hovering
in nothingness
with a secret.
(From Microbe to Consciousness)
AJKamper, the universe had an energy density far beyond 14TeV, if that's what you're driving at. Such colliders test science at the levels of energy attained early in the universe. At some point, probably, they'll break down, and when they do (even if we find nothing more) will be interesting.
Whether it's worthwhile is pretty irrelevant: we make the meaning of our lives whilst we live it.
And finding out something so the next generation can build on that knowledge is as good a thing to do as any, IMO.
Lots of comments to deal with, so, a selection in reverse order:
AJKamper @21, Fermilab went up to 2 TeV and had virtually no shot at finding a 125 GeV Higgs. The difficulty is that these protons that you're smashing together aren't single particles, but composites of quarks and gluons. So you need enough energy (in the center-of-momentum frame) between one constituent particle of each proton to produce this 125 GeV particle, and that's incredibly rare. (Less than one-in-a-billion collisions at this energy, FWIW.) As for what's next, we'll never know if we never look.
Jochem @17, we say, based on the physics we've verified and observed to date, what do we expect the event rate of XXXX event at YYYY energy to be? So if you know your expected rate (say, one-in-ten-trillion) and your number of events (say, 400 trillion), you know how many events you expect to get: 40. But what if you see 32? Or 45? Or 145? Is that indicative that something new and/or weird is going on? That's what the green and yellow shading show you. There could be a suppression at ~380 GeV and an enhancement at ~250 GeV because of new physics (seen by ATLAS), or it could be a statistical fluctuation. Why does more data help? Let's say you've now got 10 times the data. If that "32" becomes "320", you might have something. But if it becomes "386", probably not. If the "45" becomes "405", it's likely nothing, but if it becomes "506", maybe you have something. And if the "145" becomes "445", then that's an example of the signal going away, but if it becomes "1450", now you have a big deal. And that's what I think is going to happen with the Higgs.
Sascha @16 and Brenden @3, Trapit is new (and in Beta), and they're the new company I'm working with (many details coming in a few weeks). This is one of my earliest experiments trying to incorporate the good things I think it does into the site; I freely admit that I have plenty to learn about what is and isn't useful and positive about it. Feedback of the things you would like to see different about it are incredibly useful at this point. The info about wanting to identify the sites that are linked to when you mouseover is a really good one, as is your feedback on my use of their links. If you have specific things you'd like to see different about the interface, let me know; I really like the real-time news aggregation (plus the quality, diversity and lack-of-spam that it pulls), but there are certainly ways it can be improved.
Also, Brenden @3, thanks for the typo catch; it's been changed.
Eddie @14, when the individual protons get all the way up to max energy -- 7 GeV apiece -- you will be correct. For the purposes of this run, they were only at half that energy, so half your gamma factor. If that means I goofed the percentage, that's my fault.
Concerned Citizen @10, 11 and 12, your logic is flawless.
Mu @4 and AJ @1, the Higgs doesn't need to live very long. If the Higgs doesn't couple to your particle at all (like, say, a photon), then it has absolutely no mass. But the more strongly a particle couples to the Higgs, the more massive it gets. How does coupling work? Well, you don't need a real Higgs; a virtual one would do. (For example, see how this Feynman diagram works.) But this also explains why it's so hard to produce a real Higgs: you need enough energy to produce not only the Higgs, but also all the intermediaries -- like, for example, a top-antitop pair -- that lead to its production.
If we figure out what its mass is, the next thing to investigate, in the standard model, is where the Higgs' couplings come from. In other words, why the particles have the masses they do; that's currently an unanswered question. Also, if neutrinos do have mass (as they appear to), does their mass come from the Higgs, or somewhere else? There is new physics out there, and the LHC is presently our best chance of uncovering it.
Love this blog! Great explanation!
Thanks for the explanation Ethan, I never realized that heavy particles like the Higgs could have virtual equivalents that work just as well.
I always hope they find what they don't expect to because it's more fun.
Other than that, I'm indifferent about subatomic particles. It's not as if anyone can do anything about it.
Thanks for your responses. The rest of this comment is regarding Trap-It. There are good things about it, that you mention above, but I'll focus on constructive criticism.
1) The interface is a bit confusing. Why does it warp me down to some particular spot on the scroll bar? Why has it prioritized the position it chose over some of the other parts? The content all seems to be similar, so I don't really know if I'm expected to scroll through and look at it all, or if it's chosen this position for me based on something. Or maybe the position it warps to is indicated in your link, and had I gotten to it some other way, it would have started me at the top?
2) Over and above the mouse-over part I mentioned @3, I'm usually likely to click a link you provided because you have chosen that particular content to illustrate or explain some point, and I trust your direction on these topics. Trap-It is just an aggregator, though (or at least I think it is), and to me it feels like it's possible that it will choose a piece of content that actually doesn't really illustrate the point all that well. I can't be sure, because I don't get the impression that you (Ethan) are controlling the content that I'm seeing when I click the Trap-It link, even though you directed me there.
Point 2 has more to do with Trap-It's usability as a place to link to from your (or any) blog. I think it's probably rarely ideal to link to an aggregator from a blog, unless it's to illustrate some point such as "look how much stuff there is out there on this topic!". As a reader, I'd prefer that you (the blog's author) have control over where I go, rather than an aggregator's algorithm.
It is an aggregator, right? It sounds like it definitely has the potential to be a *very good* aggregator, don't get me wrong.
2 questions (with preamble & postamble)->
I'm aware that most of the mass of nucleons is from quark-quark interactions; energy is mass and a system made up of bits that push each other and move relative to each other has more mass than a system with all the same bits but the bits are stationary and non-interacting. My understanding of theory is that the rest mass of Standard Model particles is all from their interactions with the Higgs field (so the "unintuitive" potential & kinetic kinds of mass are in theory the only mass).
1) How wrong is everything I've said up to now?
2) Have we measured that gravitational potential also works this way? i.e. Will an observer far away from 2 1kg stationary balls (say dark matter balls to remove EW interactions) measure more mass when the balls are stationary and separated then when they are stationary and touching?
Obviously a "no, gravity doesn't do this" would mean that an accretion disk has more mass than the particles it's made of had before falling down its gravity well (after accounting for radiation); so the theory of energy conservation would tell me "yes". I'm hoping you can answer with something like "our models of galaxy formation don't match reality if we assume gravity is different" or "we haven't found structures that are measurably different if gravity is weird this way".
(Quick calculation puts the kinetic mass of the Earth in the Earth-Sun system at 5E-11 times the Earth's rest mass so question 2) isn't completely trivial I hope).
Dear Ethien,
at comment 25 you reply to comment 21 basically saying that Tevatron did not have enough energy to produce Higgs at 125 GeV. Are you sure? During the last years they claimed that their data excluded the possibility of a Higgs between ~150 and ~180 GeV (I do not remember the exact numbers). But how could they claimed this, if you were true and they could not have produced the Higgs there for lack of energy? In fact, I find it strange that recently Fermilab have not presented their data based on an integrated luminosity which doubles the LHC one, although collected at a lower centre-of-mass energy, and I think it would be interesting to see what they have got.
Thank you for this it is extremly interesting and informative and I will be kept occupied for many hours trying to understand it all, but I find it fascinating and want to learn and know more and with your help I will.
THANK YOU
Ethan,
I have a theoretical question:
Assume we can control the higgs field. If we could "remove" the saclar effect of the higgs on an object; would that object then accelerate to the speed of light? I guess a better way to put it is: without mass would we be like a neutrino or a photon? If yes then id say that would be one giagantic step for space travel and payload launches lol...
Jim, I would say it depends on whether inertial mass is imparted by the Higgs Boson, and whether gravitational mass is important in special relativity, rather than inertial mass.
If the Higgs boson makes the inertial mass zero, the equation for special relativity is undefined. The "divide by zero" problem is only solved for a zero-divided-by-zero dependent on how the numerator and denominator get to zero.
1/x gives infinity when x=0.
x/x gives 1 when x=0.
(as two very simple examples)
In the case of a photon, the speed of light is fine not because the rest mass of a photon is zero, but the calculation of the mass of a photon gives a zero gives a definite answer in SR mass.
There's no such need for a massive rest-mass particle disconnected with the Higgs field to get a zero rest mass that gives a valid answer, and even if such a valid non-infinite answer were gained, there's no need to assume that we'd get light speed out of it.
0/(imaginary number) is 0 if the imaginary number is not zero.
Therefore you can have an imaginary relativistic gamma and a speed faster than light.
Someone who knows maths better than me can give clearer examples.
Hi Ethan,
is it perhaps an option for you to do a follow-up about Higgs field physics, describing the things that Jim Pivarski describes in the following 2007 guest blog on The Everything Seminar:
http://cornellmath.wordpress.com/2007/07/25/the-origin-of-mass/
Rephrased in my own inadequate words Jim Pivarski expresses it as follows: interaction with the Higgs field imposes an energy cost for particles to exist at all. This energy has a corresponding inertial mass, as codified by relativistic physcis.
Jim Pivarski also describes that particle physicists are mainly interested in the Higgs field because of the implications for possible future theories.
The tidbit that makes it out to the general public (the Higgs field as responsible for giving particles mass) is not at the center of attention.
I'm sure trap.it is very nifty, but personally I find it incredibly annoying that I can't tell where a link is going until I click it.
But I hope that's just me.
Thank you for the feedback, Sili!
Trap.it is still in beta, and that issue is certainly not yours alone. (See my comment above.) Thanks for bringing it to my attention, and I'll do my best to both address it soon and improve it!
Sili, most browsers have a status bar at the bottom of the window which will highlight the link underneath the hyperlink. Many browsers also put a bubble with the link destination next to the mouse pointer.
It's up to the browser and the web page may need an ALT tag to give the browser a hint.
no God, no Higgs boson
Hello Ethan,
Thanks for writing this. It's a really well-structured explanation of the recent research results. I always turn to this blog to get well-balanced insights in topics like this.
Stefaan
I offer an explanation of a Higgs @ 124.443 GeV http://theoryofeverything.org/wordpress/?p=563
Fascinating!!