"Like all animals, human beings have always taken what they want from nature. But we are the rogue species. We are unique in our ability to use resources on a scale and at a speed that our fellow species can't." -Edward Burtynsky
It's really a romantic notion when you think about it: the heavens, the Milky Way, is lined with hundreds of billions of stars, each with their own unique and varied solar systems.
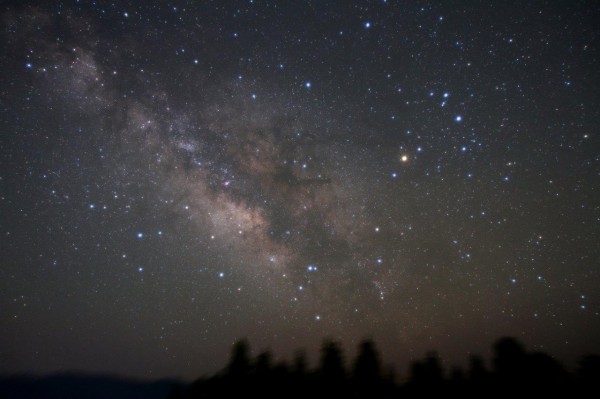
But beyond that -- in addition to the stars -- there are hundreds of billions of planets with no central stars at all: the rogue planets of our galaxy. We think this is true everywhere, from small star clusters to giant galaxies. As best as we can tell, there are at least as many starless planets wandering the cosmos as there are stars, meaning that for every point of light you see, there are probably more massive points that exist, but emit no visible light of their own.
We've recently discovered a number of possible rogue planet candidates, although since these are so difficult to detect (and are only visible from their heat signatures in the infrared) we know that there must be many, many more than what we've seen so far. You can't help but wonder where these rogue planets come from!
One compelling source is near and dear to us all.
We know how solar systems like our own form: you get a central star with a protoplanetary disk around it. Gravitational perturbations in the disk attract more and more matter from their surroundings, while the heat from the newly formed central star gradually blows much of the lightest gas away into the interstellar medium. Over time, these gravitational perturbations grow into asteroids, rocky planets, and eventually -- for the largest ones -- gas giants.
The thing is, these worlds don't just orbit their central star, they also gravitationally tug on one another! Over time, these planets migrate into the most stable configurations they can attain, and this usually mean the largest, most massive worlds migrating into their most stable configurations, often at the expense of other worlds.
A recent simulation shows that for every planet-rich solar system like our own (with gas giants) that forms, there's likely to be at least one gas giant planet that gets kicked out, into the interstellar medium, where it's doomed to wander the galaxy on its own as a rogue planet.
That's almost definitely a major source of rogue planets.
But here's the funny thing: when we work out the numbers of our best theoretical calculations, it's far less than 50% of all rogue planets that are expected to be accounted for by this process. To figure out where the majority of starless planets come from, we have to look at a larger scale at around the same time: not just when the Solar System formed, but at the cluster of stars (and star systems) that all formed at around the same time!
Star clusters form from the slow collapse of cold, mostly hydrogen gas, typically within a galaxy. Within these collapsing clouds, gravitational instabilities form, and the earliest, most massive instabilities preferentially attract more and more matter. When enough matter gets together, and the densities and temperatures at the core of these clouds get high enough, nuclear fusion ignites!
This results in new stars and star systems, but something else happens, too. The biggest stars that form are also the hottest and bluest, meaning they emit the most ionizing, ultraviolet radiation.
So when you look inside a star-forming nebula in the Universe, you are actually watching two processes simultaneously competing:
- Gravity, as it attempts to pull matter in towards these young, growing gravitational overdensities, and
- Radiation, as it works to burn off the neutral gas and blow it back into the interstellar medium.
Who will win?
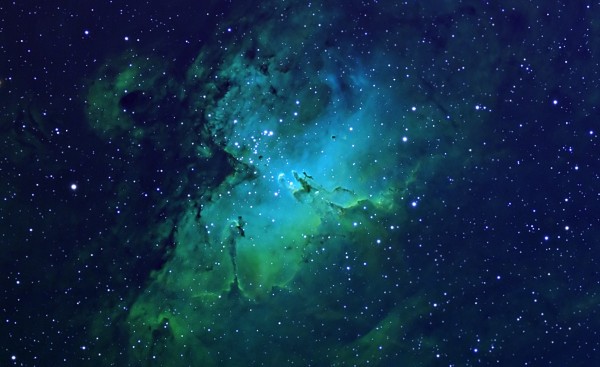
It depends what you mean by "win," exactly. The biggest gravitational overdensities form the largest stars, but these are also the rarest of all stars. Smaller (but still large) ones form the other star types, but become more and more common as we get down to lower masses. This is why, when we look deep inside a young star cluster, it's easiest to see the brightest (blue) stars, but they're vastly outnumbered by lower mass, yellow (and especially red), dim stars.
The thing is, if it weren't for the radiation, there dim, red-and-yellow stars would have grown more massive, brighter, and burned hotter! Stars (on the main sequence, which is most stars) come in a variety of types, O-stars being the hottest, largest and bluest and M-stars being the coolest, smallest, reddest and least massive.
Even though the vast majority of stars -- 3 out of every 4 -- are M-class stars, compared to less than 1% of all stars being O-or-B stars, there's just as much total mass in O-and-B-stars as there are in M-stars.
And it turns out that some 90% of the original gas-and-dust that was in these star-forming nebula gets blown off back into the interstellar medium rather than forming stars. The most massive stars form the fastest, and then get to work blowing the star-forming material out of the nebula. By time a few million years go by, there's less and less material to form new stars at all. Eventually, all the leftover gas-and-dust will burn off completely.
Well, guess what? Not only are M-class stars -- stars between 8% and 40% of the Sun's mass -- the most common type of star in the Universe by far, but there are a whole lot more that would have been M-class stars if it weren't for the high-mass stars burning off this material!
In other words, for every star that forms, there are many, many failed stars that didn't quite make it; anywhere from tens to hundreds-of-thousands of them for each star that actually forms!
These nomad planets -- or rogue planets -- may or may not have atmospheres, and they may be incredibly difficult to detect, especially the (theoretically) more common ones: the smallest objects.
So we may have a few rogue planets that were ejected from young solar systems, and there may even be a couple that came from our Solar System, but the vast majority were never attached to stars at all! Rogue planets wander the galaxy, most of them to toil forever in loneliness, never knowing the warmth of a parent star, thwarted by stellar evolution from ever becoming stars themselves. What we have, instead, is a galaxy with trillions or possibly even quadrillions of these nomad worlds, objects which we're only just beginning to discover. And that's where rogue planets come from!
- Log in to post comments
So are the masses of the Roque Planets taken into account in the tally of the mass of the Universe ? Are they part of the 4 % ? What is the best estimate for the mass difference between "trillions " and "quadrillions" ? Thanks.
Is there a reason for the power law that says that each class of stars has roughly the same total mass? It seems unlikely to be coincidence, yet there doesn't appear to be any obvious reason why there should should be any scaling law, let alone one that is so orderly.
In the hypothetical case of a gas giant booted out of its orbit by Jupiter, why is it so obvious that it would be totally expelled from the solar system? Couldn't it still remain in a very distant orbit?
Wow! Like using exclamation points, much!
Yes, a gas giant thrown into the far reaches of the solar system can get stuck there (identical to how we produce Oort cloud comets), but the chance of that happening is only a couple percent. So - probably not, unless there were dozens.
@ 1
The mass of any rogue planet compared to 1 solar mass is extremely tiny. Given a galaxy you might at best get 0.1% contribution. And yes, they are in 4% part since they are made of matter like everything else.
@2
yes, you can check stellar classification. Basically it's like black body radiation. Different colors, different temperatures hence energies, hence composition, hence mass needed to produce such and such radiation.
SL, re answer to #1, it would be more complete and helpful to say that it is part of the 4% because it will, despite being "dark", it interacts with normal matter and can be captured like normal matter. It is also able, since it is a coalesced body of significant size, be able to spot a significant portion by their occlusion of starlight.
True Dark Matter (tm) doesn't interact even with itself well and therefore doesn't coalesce and, if it reacted with photons (the idea is it would not, any more than it would with normal matter, but if it did, it would be pushed out of every stellar envelope by photon pressure), it is a diffuse body and does not cause extinction in the same way.
And like Velikovsky said, when a HUGE object (we are calling them rogue planets here) comes into the influence of a star, it becomes a comet. As Jim McCanney says, the object then discharges the Solar Capacitor like a bug zapper. This causes the Sun to begin to increase its activity. The bigger the object, the more the solar activity. We do not see HUGE comets the size of Venus too often, luckily, but we have had them pass nearby on occasion. They are usually very destructive and can account for massive Earth changes simply by passing near by, without impact. Like Velikovsky proposes, Venus was a comet before it fell into its present orbit. It happened in recorded history and caused people to go underground at the time it passed by us, possibly 4K years ago. Venus even exhibited a tail.
@ Wow
Well, I did say it :) "And yes, they are in 4% part since they are made of matter like everything else."
you must have missed it.
@3: It's possible for a planet to be kicked into an orbit which is highly elliptical but still bound. To do that, you would have to boost its speed to somewhere between the circular orbit speed and escape speed for its previous location. IIRC, these two speeds differ by a factor of sqrt(2). The problem is whether such a planet would remain in that orbit without having nasty effects on the planets remaining in more conventional orbits whenever this planet approaches periastron. Near apastron, it wouldn't take much of a gravitational kick from a nearby star to turn this planet into an Oort cloud object (similar to how Oort cloud objects become comets), or finish the job of kicking it out of the solar system. Alternatively, the remaining planets could finish the job when this planet next approaches periastron.
No, you said it but it was implied, not explicit.
All you said in explicit form was "it's not like dark matter because it's like the regular matter".
You never explained why it would be known to be part of the 4% of normal matter and not part of the 20-ish% of dark.
Just said it wasn't.
true, it was implied, because Ethan's article is about it. Since they are made of the stuff that was in our solar system.
Didn't think @1 was asking if they were DM, rather if their mass contribution was accounted for in normal matter. Guess it's how you interpret the question. Anyways you added more to the answer so it's all cool :)
"Since they are made of the stuff that was in our solar system. "
sorry.. should be our/other solar system.. hence hydrogen, rocks, metals etc...or just gas giants
"Didn’t think @1 was asking if they were DM,"
That's how I read it.
Use the term rogue planet and the cranks come running in, as displayed by wally58 above.
I believe the theoretical argument that they should form, but what direct observation is there that they do form?
@16
not likely to get "direct observation" any time soon. Think about it. How do you observe a tiny speck that doesn't emit light.. hence black.. against a black background of space?
It will be a major breakthrough when we get to "directly observe" any planet outside of our solar system. Those wandering the blackness of space.. who knows.. maybe in some other bands if they emit something.
"How do you observe a tiny speck that doesn’t emit light.. hence black.. against a black background of space?"
I'm reminded of the conversation aboard Hot Black Desiato's ship with this...
I believe a few months ago we have the first direct observation of an extrasolar planet.
Though that might have been the observation of the absorption spectra of a planetary atmosphere (dense enough neutral matter to be neither space dust nor stellar atmosphere).
@Wow #19: Direct observation of extrasolar planets goes back several years, to at least 2004. HR8799's four planets were imaged back in 2010; AB Pictoris has a companion which is either a brown dwarf or Jovian-mass planet, directly imaged in 2003. See http://en.wikipedia.org/wiki/List_of_directly_imaged_extrasolar_planets for a current list.
Thank you Michael! This is awesome. To this day I wasn't aware that this was done.
Read the paper on GJ 504. Awesome! A real image of a planet orbiting some other sun. So cool!
p.s. Ethan, why no article on that we are actually able to image exoplanets?? :D Bad Ethan!! :))
Well, I remembered a story a few months ago (earlier this year anyway) and may have been about the direct measuring of the atmosphere of the planet rather than imaging the planet directly.
Rogue planets came from bad family backgrounds?
@Wow #23: Ah! I think you're remembering the recent spectroscopic analysis of HD 189733b (http://en.wikipedia.org/wiki/HD_189733_b).
A study of the combination of absorption features derived for the planet from secondary eclispes allowed the authors to "estimate" the apparent color the planet would have if we could see it directly.
No exoplanet has yet been imaged in "true color," although, given the progress in the field, that is only a matter of time.
Probably is, Mike.
Everyone: ferocious criticism invited for the following:
Are there any estimates of (or any reasonable basis for estimating) the occurrence of approximately-Earth-mass rocky rogue planets?
Those might be useful for interstellar colonization as follows:
1) Locate Earth-sized rogue planet.
2) Build Dyson rings around two or more of its nearest suitable stars.
3) The Dyson rings capture energy from the stars and convert to microwave laser or similar means, directed toward artificial moons (or captured space rocks with infrastructure built) orbiting the rogue planet.
4) At the moons, incoming energy is converted to a form that can safely be beamed down to the planet's surface and used.
5) Meanwhile, the Dyson rings are inhabited by minimal populations needed to maintain them. These should be small enough populations as to easily migrate off the Dyson rings to the client planet when the stars became hazardous toward the end of their useful lives.
Why go through all that effort, when there are Earth-sized planets orbiting stars, that can be colonized?
Because then you have an inhabitable world that is not a) dependent on and b) at risk from, a single star. Its usable lifespan would be equal to the useful life of the most long-lived of the stars that provided it with energy. Assuming you need at minimum two stars to supply energy to a rogue planet, build Dyson rings around a total of three or more stars, and you have time to replace any of them that are lost in stellar explosions.
Also it would seem that rogue planets in and of themselves, could potentially have the raw materials needed to support life, but be lifeless due to lack of energy sources. Thus they are ready to colonize with zero to minimal risk of encountering indigenous microbes that could prove fatal.
Comments?
G: even for sci-fi, your proposal is extremely Rube Goldberg-ish. Any civilization that can do step 2 has no need of any of the other steps; it can simply move to another system when the old star is close to burning out.
Secondly, wouldn't it just be easier to locate a rogue planet with some internal heat associated with it, and just use that? Whether gas giant or rocky, the rogue planet's gravitational force on its own mass will tend to heat things up at depth. I have to think that extracting that heat as work must be a great deal easier than your set-up. A jupiter-sized geothermal generator is (speculatively) peanuts compared to ringworlds firing interstellar lasers.