“There’s no god, it’s the elements that control this world and everything on it.” -Scott A. Butler
From hydrogen through uranium and even beyond, the Universe gives us a huge variety of elements that can bond together in practically innumerable ways, creating all the matter we've ever observed in existence. Everything beyond helium in the periodic table way made inside of stars, but not all stars create elements equally.
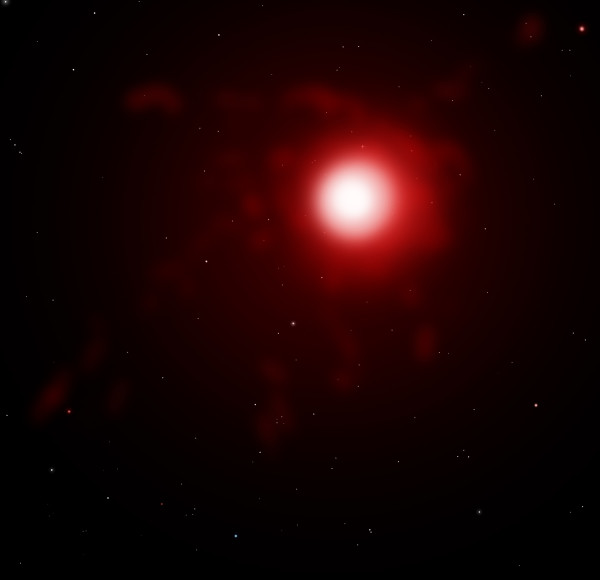
Our Sun will someday become a red giant, fusing not just helium into carbon, but creating elements that rise higher and higher in the periodic table. Yet there's a limit to what even a red giant can create, as there are some elements that require a process our Sun will never know.
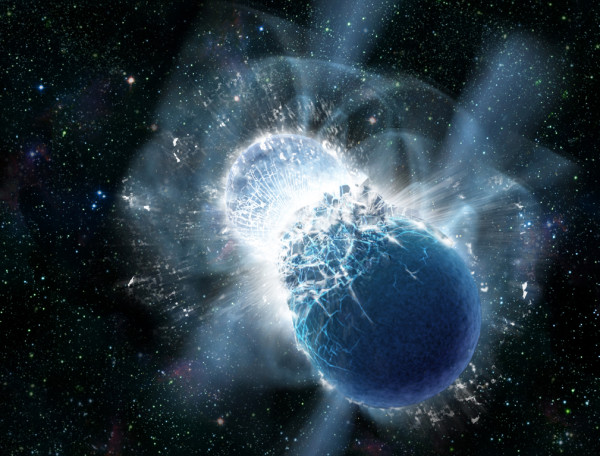
Find out which elements the Sun can (and cannot) make today!
- Log in to post comments
@Ethan: You wrote, "Even the very first core-collapse supernovae created [via the r-process] elements that are beyond the limit of what we find on Earth: elements heavier than even uranium and plutonium." I've got two questions about this, one theoretical and one observational.
Theoretically, how confident are you that the r-process (i.e. single neutron capture) can really produce trans-plutonian elements? Most of the decay channels for those (man-made) elements are alpha decays, not betas. I couldn't find (in my cursory search) papers to support your statement; any chance you can point me to a decent review?
Second, are there any plausible observational results (gamma lines, for example, or even neutrino "lines") that one might possibly see in the first few seconds of a supernova, which would reveal superheavy actinides? Given that their decay modes are primarily alphas, I would guess not, but nuclear structure isn't my specialty.
@Ethan: Still on forbes? I don't understand. Something is rotten in the state of Science.
I'm guessing that for either the S or the R process, that each step of these long chains results in lower abundance as you climb to higher atomic mass. Presumably the slope of the log of abundance versus mass is pretty steep, and probably pretty dependent upon details of the state of matter (pressure/temperature history mainly). Can reasonable estimates of the relative abundance contributions from the various mechanisms be made?
@Ethan
Will you publish my comment? Is there anything wrong with it? I mean, you are a scientist, you are on facts, aren't you.
Hi Michael. I don't have any cites handy but I think I can give you at least a qualitative answer to your questions. AIUI the r-process isn't just single neutron capture, and it's the multiple-N captures that eventually produce the heaviest elements (add 10 neutrons, then beta decay, rinse and repeat).
Since the probability of a multi-N capture decreases with number of neutrons, we would expect in general that the amount of high Z elements produced will fall off with Z. However the actual numbers are going to depend on neutron flux, capture cross sections of intermediates, stability of those intermediates, etc.
My initial expectation is no or at least not right now. Most of these reactions are going to produce highly excited nuclei so we could expect some gamma emissions from them. However, again that decreasing probability works against us, because it means we're going to have lots of noise (i.e. gammas from other reactions) compared to signal (gammas from transactinide-producing reactions). There is also the problem of not knowing (with high confidence) what is 'signal' in the first place. Though hopefully experiments that produce transactinides can help nail some nuclear structure uncertainties down, and thus be useful for astronomers who want to study the limits of the r-process in supernovae.
@eric #3: Thanks, eric! I know that for most of the r-process, you're quite right that heavy-element production runs via multiple neutron captures (basically, it runs up the neutron drip line). But the transactinides have such short half-lives (milliseconds or less), that I wonder whether you'd be able to drive multiple captures before decay.
As an add-on to my last comment: probably the limit of what we could do now is take a supernova spectrum, subtract out all the known signals, and then see if any remaining lines match up with theoretically calculated de-excitation gammas in high-Z isotopes we haven't studied in the lab. However, that would (IMO) be just the first step on the way to saying we've 'discovered' a new isotope or 'demonstrated' their production. To make those conclusions with high confidence we'd want some lab-based experiments showing that the decay in question does indeed have the gamma line we see in the supernova spectrum.
Well its all relative. Milliseconds is short for humans but Wikipedia tells me the flux of neutrons in a supernovae is 10E22 per cm2 per second. An isotope "only" lasting a millisecond has plenty of time to suck up neutrons.
@eric #8: Hmmmm. This reminds me of Ethan's comments on my flux calculation for the interstellar solar sail....
So, a neutron flux of 10e22 cm^-2 s^-1 corresponds to 0.01 barn^-1 s^-1. Since neutron capture cross-sections are typically a few barns (which is how that unit was invented, after all :-), that suggests a capture rate of well under one neutron per second on a given nucleus.
So to get r-process elements beyond plutonium, you need half-lives of several minutes, if you want the nucleus to hang around long enough for multiple captures. Let's see...
Plutonium has half-lives measured in years, even for the short-lived ones, as do actinides up through curium. Beyond that, the actinides up through nobelium half half-lives of days, and up to borhium (Z=106) there are isotopes with half-lives of hours or minutes. Beyond that, the half-lives drop to seconds or less.
So I misspoke. Not all the transactinides have sub-second half-lives, which means some of them are, as you've said, produceable with supernova-scale neutron fluxes. But as you pointed out in #7, actually pulling a signal out of SN prompt X-ray or gamma-ray would be quite a trick!
"Hi Michael. I don’t have any cites handy but I think I can give you at least a qualitative answer to your questions. AIUI the r-process isn’t just single neutron capture, and it’s the multiple-N captures that eventually produce the heaviest elements (add 10 neutrons, then beta decay, rinse and repeat)."
Additionally the neutrons would be close enough for the formation of resonances with each other, retaining them in the region for a while longer. This is more likely around mid-level (Oxygen generation) novae processes where the temperature is still "fairly" low but the density of the object still fusing high.
It's not common, though.
But when you've got 10^40 atoms piddling about, a one-in-a-million chance happens really often...