“We are not simply in the universe, we are part of it. We are born from it.” -Neil deGrasse Tyson
The story of the Universe is the story of us all; we all share the same cosmic history, coming from a hot, dense state some 13.8 billion years ago known as the Big Bang and emerging after billions of years of cosmic evolution to the Universe we know and love today.
It's a beautiful story -- and one I've told before -- but it might seem, at least from our perspective, that something is missing from the astrophysicist's version of events. Yes, we can start with a hot, dense, expanding Universe full of matter, antimatter and radiation, create some asymmetry between matter and antimatter, and then have the Universe expand and cool. Below a certain temperature, the antimatter will annihilate away with the vast majority of the matter, leaving only the tiny, asymmetric amount of matter (less than 1-part-in-a-billion) bathed in a sea of radiation.
But this radiation is constantly dropping in temperature as the Universe expands, having its wavelength stretched by the expansion of space. At some point, the Universe becomes cool enough that two types of matter particles you know -- protons and neutrons -- can fuse together without being immediately blasted apart by this sea of radiation.
These nuclear fusion reactions give it all they've got, but by time this can happen, it's very difficult to get densities (or energies) high enough to form very heavy elements. During the first few minutes after the Big Bang, we can create a substantial amount of helium in the Universe, and tiny, trace amounts of Lithium and Beryllium, but that's it. The story of Big Bang Nucleosynthesis is amazing, but it doesn't tell us where we come from.
By number, it gives us a Universe which is 92% hydrogen, 8% helium, and less than 0.0000001% everything else combined. In fact, if we want the elements we consider essential to life -- Carbon, Nitrogen, Oxygen, Phosphorous, etc. -- we have to look well past the early stages of the Universe, and fast-forward to the formation of stars!
The more massive your star is, the heavier the element it's capable of fusing together. Also, the more massive your star is, the faster it burns through its fuel! A star like our Sun will fuse helium into carbon, nitrogen, and oxygen, while a substantially more massive star like Sirius (the brightest in our sky) will fuse those elements further into silicon, sulphur, and all the way up to iron, nickel and cobalt. When these stars run out of fuel, they'll return a fraction of these heavy elements back into the cosmos when they expel a large fraction of their mass into a planetary nebula.
But the elements heavier than that -- as well as the vast majority of all elements heavier than helium -- come from the catastrophic deaths of the most massive stars: the one that die in core-collapse supernovae! Whenever a star more than about 8-to-10 times the mass of the Sun is formed, it burns through all of its nuclear fuel in just a couple of million years, maximum, and its core collapses down to a neutron star or black hole, destroying the rest of the star. Large amounts of the first 28 elements of the periodic table are recycled back into the Universe, where they can form the next generation of planets and stars.
But a huge number of free neutrons are also created, and through two creatively named processes -- the rapid r-process in the supernova itself and the slow s-process in the later stages of these stars' lives -- the heavier nuclei are created, including everything up-to-and-including Plutonium, and probably even beyond!
Yes, these elements will continue on in the next generation of stars, planets, and whatever else happens to come to exist in the Universe, making up as much as 1% of the Universe by mass (although much less than that by number). But there's a long way to go from atomic nuclei to the world we know today. And that connection goes far beyond my expertise. Luckily, I've got a very smart friend whose expertise lies in chemistry (and who has guest blogged for us before), and he's agree to take us through some of the basics of astrochemistry, or how these elements bind together to form molecules in the Universe. [My comments in square brackets.] Take it away, Richard Helmich!
Thanks Ethan for the excellent lead in and introduction. From the many generations of stars, the Universe has generated plenty of elements, and humans have even taken their time to organize them into the periodic table. These are very same elements found in you and me, and out in space these atoms don’t simply float around and do nothing. They react with each other to form all kinds of familiar and exotic molecules. Let’s take a look at what chemicals we’ve found outside of Earth so far…
Hang on a minute… what are astrochemists looking for, exactly? Similar to how atoms absorb and emit light characteristic to a particular element in planetary nebulae like the Ring Nebula, whole molecules can absorb energy and emit light of particular frequencies characteristic to that molecule.
The wavelength of light that a molecule absorbs or emits depends on what the atoms in the molecule are doing before and after the light is absorbed or emitted. The atoms in the molecule can vibrate in relation to each other in various ways, or the whole molecule can start or stop spinning as a whole.
Symmetrical stretching |
Asymmetrical stretching |
Scissoring |
---|---|---|
![]() |
![]() |
![]() |
Rocking | Wagging | Twisting |
![]() |
![]() |
![]() |
The study of the energy atoms and molecules absorb and emit is an entire field of science called spectroscopy. Scientists have been able to discover the identities (and sometimes even the concentrations) of molecules that form throughout the universe by using spectroscopy to examine the infrared and millimeter wavelength light emitted by molecules in outer space. The amount of light given off at these wavelengths is typically very small, but fortunately scientists have been able to build very powerful telescopes such as the Herschel Space Observatory, Spitzer Space Telescope and -- here on Earth -- the Atacama Large Millimeter/Sub-millimeter Array (ALMA) to investigate astrochemistry in the nearer parts of the universe.
So what have scientists found out in the vast expanses of space so far?
LOTS!
Here are just a few that have been identified, that are also common on Earth. (And there's a more complete list here.)
A few molecules found in outer space. |
||||
2 atoms |
CO Carbon Monoxide |
NaCl Sodium Chloride |
NO Nitrogen Monoxide |
CS Carbon Monosulfide |
3 atoms |
H2O Water |
HCN Hydrogen Cyanide |
H2S Hydrogen Sulfide |
FeCN+ Iron (II) Cyanide |
4 atoms |
C2H2 |
NH3 Ammonia |
H2CO Formaldehyde |
H2O2 Hydrogen Peroxide |
Others |
CH3OH Methanol |
SiH4 Silane |
CH3CHO |
C60 Buckminsterfullerene
|
So let’s follow what happens to the elements created as a star dies and then are recycled again into a new star…our first stop is IRC +10216.
This star was once between 3 and 5 times the mass of our sun, Sol. This star is in the late stages of becoming a white dwarf, and is in the process of blowing off its outer most layers into interstellar space. An astute scientist studying this star noticed it had a plethora of chemicals and was even able to map the location of some of them.[1]
This map is awesome! Some scientists are figuring out various ways to do this for very small objects located on Earth, while others have mapped the molecular makeup of a star hundreds of light years away. [This star, in particular, is some 400 light-years distant from Earth.] This map has given scientists insight into the evolution of this star during its dying moments.
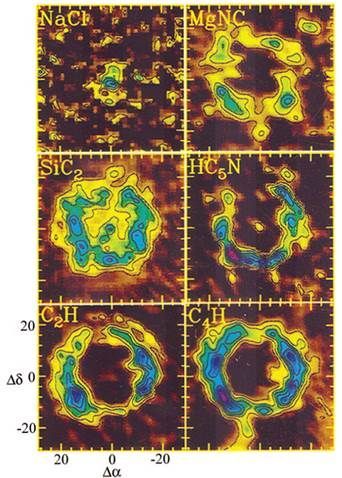
The NaCl (the same as the salt you have in your kitchen, minus the added iodine) is only observed near the core where temperatures are hot enough to keep it in the gas phase. Farther away there is likely to still be NaCl, but it has cooled and condensed into dust and is no longer obserable. Magnesium isocyanide, MgNC, is another metal salt like NaCl. This molecule is peculiar because on Earth the magnesium atom would be bound to the carbon (MgCN), but the reaction in outer space is kinetically driven (molecules and atoms bumping into each other) not thermodynamically determined so typically the abundance of each isomer is approximately equal.[2] Other molecules were also observed such as HC5N and C4H. These are acetylenic molecules (similar to acetylene used in welding torches) with alternating single and triple bonds between the carbons atoms.
Later, after the star has proceeded further along its path towards becoming a white dwarf, the amount of UV light it emits increases, forming a planetary nebula when the gases become ionized. The UV light given off by the newly formed white dwarf typically causes much of the previously formed gaseous molecules to decompose, but recently scientists have observed that some molecules are still able to hold together.
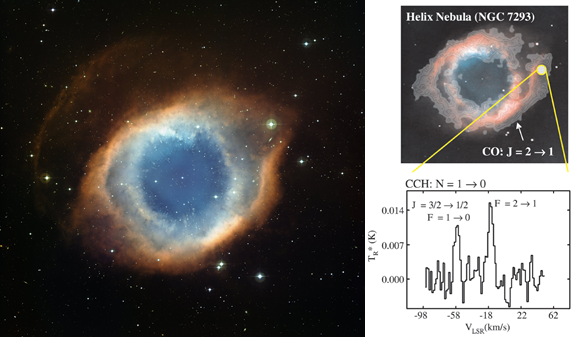
Moving from the death of stars to the birth of the next generation of stars, other scientists have observed the chemicals present in molecular clouds as they condense and form new stars. Scientists have observed water, carbon dioxide, silicates (similar to the grains of sand found on any beach), and even polyaromatic hydocarbons (also found in crude oil).[3]
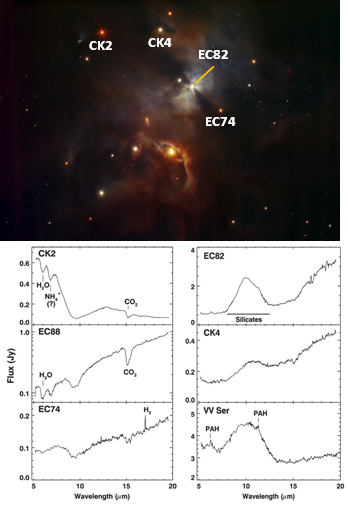
This group of scientists observed the molecular fingerprints of many newly forming stars before stumbling on protostar IRS46 in Ophiuchus. The protostar showed a very interesting mix of acetylene (C2H2), hydrogen cyanide (HCN), and carbon dioxide (CO2) gases.
None of the other protostars observed had these gases, possibly because IRS46 is oriented with it’s planet forming plane directly along Earth’s line of sight. Observing these gases around protostars is very exciting, because they are some of the most basic building blocks of protein and DNA! [Other protostars, such as HH46 IRS, offer some spectacular absorption features of some common molecules as well in their outflows.]
So there you have it, a taste of astrochemistry! Our universe is full of fantastic stars that are fusing heavier elements from the hydrogen and helium created during the Big Bang and recycling them into supernovae and planetary nebulae, more stars, planets, and even you and me. When you look up into the night sky now, I hope you’ll keep in mind that while there’s a lot of hydrogen floating around out there, there’s also much, much more.
BONUS: “BUT WAIT! How do these reactions happen when the universe is generally so COLD?!”
Good Question! Some scientists recently suggested quantum tunneling might be the answer.[4] If you get a smirky ‘cool’ smile anytime quantum tunneling is mentioned, don’t worry, I do too. Let me take a second to explain more clearly what quantum tunneling is, exactly. Quantum tunneling is a process where a very small portion of many particles are able to tunnel through an insurmountable energy barrier instead of traveling up and over the energy barrier. So what does quantum tunneling have to do with chemistry in interstellar space? Well, every chemical reaction has some energy barrier between the reactants and products. This energy barrier is called the activation energy of the reaction. Sometimes the thermal energy at room temperature is enough of a kick for a reaction (acid-base reactions), while other reactions require a much harder push (a spark to ignite the gas in your car’s engine).
Back to astrochemistry, among the more common molecules found in the universe are methanol (CH3OH) and hydroxyl radicals (·OH). These chemicals react to create a methoxy radical (CH3O·) and water. All of these have been observed in outer space, but the activation energy required for this reaction was thought to effectively prevent it from happening at the temperatures found in outer space, ~ 20 K. No one had actually attempted to measure how well these chemical reacted with each other at temperatures approximating those in space. Until now!
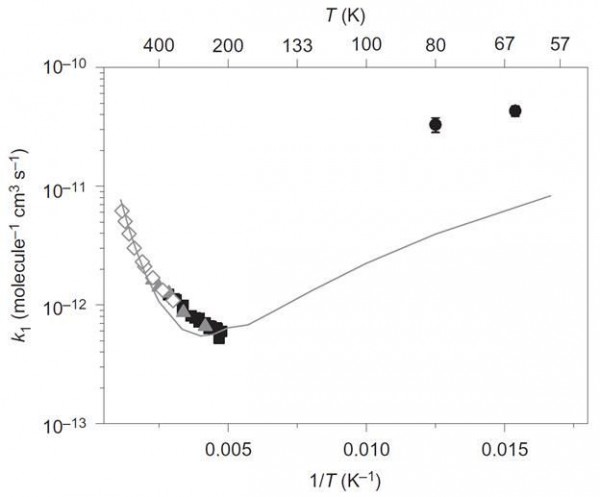
This graph shows the reaction rate constant for methanol reacting with hydroxyl radicals. Data on the left are historical and show the thermodynamic reaction path. As the temperature decreases, the rate of the reaction also decreases, but those two points on the upper right (2σ-shown) show that at even lower temperatures, the rate increases. One of the ways for a chemical reaction to form products faster than the thermodynamic path (and the most likely culprit for this reaction) is for quantum tunneling to occur. The solid line is the combined thermodynamic and quantum tunneling best fit computer model.
The Universe is an amazing place to live, and nature always finds a way to do what it wants. Even if that means a little cheating via quantum tunneling. ☺
References:
- Ziurys, Lucy M. The chemistry in circumstellar envelopes of evolved stars: Following the origin of the elements to the origin of life Proceedings of the National Academy of Sciences2006, 103, 12274-12279.
- Klemperer, William Interstellar Chemistry Proceedings of the National Academy of Sciences of the United States of America 2006, 103, 12232-12234.
- Dishoeck, Ewine F. Chemistry in low-mass protostellar and protoplanetary regions Proceedings of the National Academy of Sciences 2006, 103, 12249-12256.
- Shannon, Robin J.; Blitz, Mark A.; Goddard, Andrew; Heard, Dwayne E. Accelerated chemistry in the reaction between the hydroxyl radical and methanol at interstellar temperatures facilitated by tunnelling Nat Chem, advance online publication.
- Log in to post comments
Thanks so much for the guest post. That was awesome.
Thank you Richard and Ethan
I hope this isn't too off topic... In the period just before the formation of the first suns [380M years after BB] could there have been all the elements present, but in absolutely minute quantities for those above Be-8? I'm thinking that there must have been instances of fortuitous collisions between atoms where the energies were sufficient to form minuscule amounts of the elements B, C, N, O etc without processing through stars
Just a thought...
Michael,
According to my calculations, Beryllium-9 is a pretty hard limit. The Coulomb barrier becomes very large and the Universe is pretty cold by time Helium forms, so while it's conceivable that you might get lucky and wind up with a few atoms of Boron, I don't see how Carbon-and-higher will be formed. That is to say, there are only around 10^80 atoms in the observable Universe, and the odds of forming Carbon (or higher) during Big Bang Nucleosynthesis is somewhere in the vicinity of less than 10^-80, probabilistically.
The universe would have more atoms in it before inflation took them away, Ethan.
Though that would have to be a hell of a lot more to get *Carbon* forming, since the energies aren't appropriate until you add a million degrees of heat in the atmosphere of a large sun to make up the difference.
But it could give a chance of an over-production of Helium making Carbon possible.
Hyper-stars would be an easier option and may be the only (if extremely rare) type of star that could form that early in the universe.
A prof at my university has some pet theories about that, never took it far enough to put limits on the options made possible, though.
Maths beyond ten fingers is hard... :-)
Very interesting post. I find it mind-blowing that detailed knowledge of the chemical constituents of such remote astrophysical bodies can be gleaned from astronomical instruments. To find evidence of the molecules that make up building blocks of proteins and DNA, already formed around nascent stars -- that's stupendous stuff. Part of me feels that people should be shouting this stuff in the streets -- but my family would dismiss that as the geek within me talking. Keep up the good work on this excellent blog.
Really awesome post !!! a taste of real chemistry behind dark science.
A very fascinating book giving the history (and physics) of these discoveries is one by Marcus Chown entitled "The Magic Furnace (The search for the origin of Atoms)"
"a taste of real chemistry behind dark science."
If you want a real "taste" of chemistry, how about this: The center of the milky way tastes like raspberries and smells of rum! Think I'm lying? Look it up!
I've seen reports recently on how neutron star collisions, as a hypothetical source of short-duration gamma ray bursts, may be responsible for quite a bit of the synthesis of "neutron rich" heavy elements (e.g. gold). How significant this is compared to say "standard(?)" nucleosynthesis via supernovae?
http://arstechnica.com/science/2013/07/making-heavy-elements-by-collidi…
love your site .